Urine-Derived Stem Cells With The Capacity for Regeneration and Differentiation: The Importance of Telomerase Activity
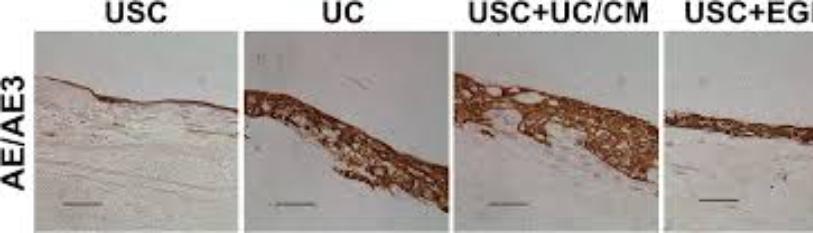
Taylor Blake1, Vincent S. Gallicchio2*
1Department of Biological Sciences College of Science Clemson University Clemson, South Carolina 29636
2Department of Biological Sciences College of Science 122 Long Hall Clemson University Clemson, SC 29636
*Corresponding author: Vincent S. Gallicchio, Department of Biological Sciences College of Science 122 Long Hall Clemson University Clemson, SC 29636.
Citation: Blake T, Gallicchio VS. (2022) Urine-Derived Stem Cells with the Capacity for Regeneration and Differentiation: The Importance of Telomerase Activity. J Stem Cell Res. 3(3):1-15.
Received: August 5, 2022 | Published: August 26, 2022
Copyright© 2022 genesis pub by Blake T, et al. CC BY NC-ND 4.0 DEED. This is an open-access article distributed under the terms of the Creative Commons Attribution-NonCommercial-No Derivatives 4.0 International License., This allows others distribute, remix, tweak, and build upon the work, even commercially, as long as they credit the authors for the original creation.
DOI: https://doi.org/10.52793/JSCR.2021.3(3)-40
Abstract
Former stem cell research and clinical application has relied on stem cells derived from bone marrow, blood, or organ-specific biopsy that requires invasive and painful procedures to obtain the stem cells. However, there are stem cells in human urine that can be used for research and potential application. This only requires a urine sample, which provides a large quantity of stem cells and good quality stem cells. These urine-derived stem cells have been used in research since the initial discovery in 2008. They can be used for cell therapy, disease modeling, and pharmacological testing. This article focuses on the most recent study from the Wake Forest Institute for Regenerative Medicine regarding urine-derived stem cells and telomerase activity. It examined whether or not telomerase activity affects the regenerative potential in urine-derived stem cells. The study found that positive telomerase activity stem cells had a higher population doubling and a shorter doubling time. Positive telomerase activity cells had strong positive markers for cell differentiation in osteogenic, smooth muscle, and urothelial cell lineages. There were not as many differences between positive and negative telomerase activity in their surface markers except positive telomerase activity cells had more cells with a strong positive expression of CD105. The study showed that urine stem cells did better overall if they were from younger donors and if they had positive telomerase activity. Lastly, the chromosome stability and tumor formation assays had the same results regardless of telomerase activity.
Keywords
Stem cells; Urine; Telomerase; Regenerative medicine; Cell therapy.
List of Abbreviations
ASCs: Adipose derived stem cells; CD: Cluster designated protein; CRISPR-Cas9: Family of DNA sequences used for gene editing; DMBT1: Deleted in malignant brain tumor 1; ESCs: Embryonic stem cells; HEK: Human embryonic kidney cells; H&E: Hematoxylin – Eosin; HLA: Human leukocyte antigen; HSCs: Hematopoietic stem cells; iPSCs: Induced pluripotential stem cells; IVF: In vitro fertilization; MSCs: Mesenchymal stem cells; MTS: Colorimetric Method; PCR-ELISA: Polymerase chain reaction-enzyme linked immunospecific assay; TA-: Negative telomerase activity; TA+: Positive telomerase activity; TGF-Beta1: Transforming growth factor beta1=SOX-1 Nuclear transfer factor; USCs: Urine-derived stem cells
Introduction
Another type of stem cell used in research is embryonic stem cells (ESCs). These cells are found in the inner cell mass of the blastocyst. The blastocyst is formed a few days after the fertilization of the egg and sperm. It contains the outer cells and the inner cell mass. The outer cells form the placenta and umbilical cord, and the inner cells form the embryo. In order to form the embryo, the inner cell mass will eventually form the three germ layers – endoderm, mesoderm, and ectoderm. ESCs have the most potential in research because they are pluripotent and can give rise to all the cell types in the body except for the umbilical cord and the placenta since those are derived from the outer cells of the blastocyst. However, there are ethical issues and concerns since these cells come from what will form an embryo. One way research facilities have been able to obtain this type of stem cells is from in-vitro fertilization (IVF) clinics. Patients going through IVF can give consent to donate the embryos that are not used to research. Yet, it is still in limited supply for research purposes along with the primary ethical concerns [2].
There is an alternative origin for stem cells which is in human urine. One can obtain adult somatic stem cells from the components of urine. This is a great source of stem cells since it obtained from a urine sample which is painless, and there is an endless tangible supply. Urine is 95% water, and the other 5% is a variety of other molecules. It contains organic molecules such as urea, creatinine, and uric acid. It contains ions like sodium, potassium, chloride, magnesium, and calcium. Additionally, it has ammonium, sulphates, phosphates, red blood cells, white blood cells, and bacteria [3]. It extremely vital to separate the stem cells from all the components of urine especially bacteria so that there is no contamination. Researchers have certain steps they follow in order to generate sterile stem cells from human urine. The first step is to add penicillin or streptomycin to the sample to reduce impurities. Then, they put the sample into a centrifuge which will spin the sample rapidly to separate the components of urine. Next, the supernatant is discarded, and they will use an anti-bacterial wash to avoid contamination. It is important after centrifuging the sample to be able to identify the separated components. According to the International Society for Cellular Therapy, MSCs can be recognized by the following indicators: 1) the cells stick to plastic culture bottles; 2) a minimum of 95% of cell population expresses particular surface antigens, including CD105, CD73 and CD90, but do not express CD45, CD34, CD14, CD11b, CD79α, CD19 or HLA-II antigens; and 3) the cells are able to be differentiated into chondroblasts, adipocytes, and osteoblasts. The final step is plating the cells in a medium for culturing [4].
After isolation of the stem cells, the next steps can be different depending on what type of cells the researchers need to work with. Certain cells that are a part of the genitourinary system would not need to be differentiated into completely different cell types. However, there is a method to create multiple different cell types from urine-derived stem cells. One of the main techniques for the utilization of urine-derived stem cells is turning those urine derived cells into induced pluripotent stem cells (iPSCs). These cells are formed by taking somatic adult cells and inducing the expression of genes to make the cell act like ESCs. This allows for stem cells to form into multiple different lineages and can be used for regenerative medicine and disease models. It can additionally be used for pharmacological testing, which is where those differentiated cells are treated with different drugs and used to test drug effectiveness and toxicity [5]. Much of the research, up until now, have utilized this method of turning the cells into iPSCs because of the ability to create various cell lines. The use of urine-derived stem cells allows for an abundant supply of stem cells that is painless and easily obtained from donors.
History of Research
Urine-derived stems cells were not recently discovered. The initial discovery was in 2008 with a study that focused on using autologous cells for the use in urological reconstructive procedures. The initial issue that sparked the study was the invasive procedure necessary to preform urinary tract reconstruction using cell therapy. It required invasive tissue biopsies in order to obtain autologous cells, and the procedures are associated with potential complications. They wanted to know if urine contained cells that had the characteristics of normal bladder cells. The researchers collected urine samples from 15 healthy individuals and 8 people with vesicoureteral reflux, which is a disorder where urine flows backward from the bladder to one or both ureters and occasionally flows backwards to the kidneys [6]. The stem cells were isolated and tested for progenitor and differentiated cell specific markers using immunofluorescence, flow cytometry, and western immunoblotting. The results showed that a subpopulation of isolated cells from urine had progenitor cell characteristics and had the capability to differentiate into several bladder cell lineages. These progenitor cells had the ability to differentiate into urothelial, endothelial, interstitial, and smooth muscle cells and, therefore, can be used as an alternate source of stem cells for urinary tract tissue engineering and reconstruction [7].
Then, in 2013 it was discovered that the telomerase activity and length of the telomeres had something to do with the expandability of urine derived stem cells. They found that one singular urine-derived stem cell possesses multipotent differentiation capability and that one urine-derived stem cell with telomerase activity differentiated into endodermal, mesodermal, and ectodermal lineages [8]. The fact that these cells can differentiate into cells from all three germ layers was a huge takeaway and the correlation to the positive telomerase activity. They also discovered that a single cell could form 5-7 clones per 100 milliliters of urine in 2-3 days which would then expand to a large number of urine derived stem cells [4]. In 2016, it was discovered that urine-derived stem cells exert therapeutic effects through utilizing paracrine mechanisms that can be initiated by extracellular vesicles such as exosomes and micro-vesicles. This study was specifically focused on treating diabetic nephropathy which results from damage to blood vessel clusters in the kidneys [9]. This is a common complication in patients with diabetes and can lead to end-stage kidney disease. The study was researching a treatment for diabetic nephropathy using urine-derived stem cells. They found that exosomes from conditioned medium of urine-derived stem cells have the potential to inhibit kidney injury from diabetes by preventing podocyte apoptosis and encouraging vascular regeneration and the survival of the cells. These exosomes displayed a paracrine pathway rather than a direct effect responsible for stem cell damage repair [10].
In 2017 researchers started to reprogram urine-derived stem cells into iPSCs. However, this came with some disadvantages. IPSC sare genetically insatiable and can lead to forming tumors or teratom as in the body if implanted. This study was done to find a way to induce the urine-derived stem cells that does not have as many complications and negative side effects. They developed a safer technique based on an episomal vector for inducing iPSCs from human urine-derived stem cells. It did not include using tumorigenic factors such as SV 40-LT, c-Myc, and p53 inhibitor. The study also showed that this specific method had a lower amount of chromosomal variation that occurred during iPSC generation than the traditional method, which reflects a better method for use in clinical application [11]. It was mainly used for endocrine and digestive cells, and it proved to rapidly proliferate and proficiently differentiate into many cell types with greater yields as well as fewer cases immunological rejection.
Thereafter, in 2018 researchers learned that urine-derived stem cells can be used for cutaneous regeneration, wound healing, and reduce the effects of osteoporosis. The first study in 2018 was primarily focused on chronic non-healing wounds that is a very common complication of diabetes. This study expands upon the use of exosomes and paracrine action of urine-derived stem cells for tissue repair and regeneration. Their findings shown that the exosomes can augment the properties of skin cells and accelerate wound healing in diabetic mice. They stimulate angiogenesis by transferring the DMBT1 protein [12]. Additionally, a study was done using iPSCs from urine-derived stem cells for treatment of ventricular septal defects, which can lead to heart failure especially in pediatric patients. They found that the protocol that they used generates an ample number of myocardial cells and displayed spontaneous contract[13]. This provides a solid platform for further investigation for the treatment of ventricular septal defects. In 2019, a treatment for osteoporosis was studied using human urine-derived stem cells. They used urine-derived stem cells that had MSC-like properties to obtain extracellular vesicles. The researchers determined that the systemic injection of urine-derived stem cell extracellular vesicles did inhibit bone loss and increased bone strength in osteoporotic mice because of their dual effects on bone metabolism. This showed to not only enhance osteoblastic bone formation, but it also reduced osteoclastic bone resorption [14].
Moreover, in 2020 those exosomes that were discovered to have a para crine function were also found to have the potential to enable angiogenesis and encourage cell survival through the production of growth factors and a transforming growth factor – Beta1 (TGF-1) [4]. Finally, it was shown in 2021 that urine-derived stem cells to iPSCs can be used for disease modeling due to the unlimited number of cells that have the same genes as diseased cells. Disease models are used to mirror a disease and show the disease progression. This allows researchers to study the role of specific genes in different diseases. Many disease models in the past used animals; however, they are not always accurate in mirroring the specific gene expression and physiology that would be displayed in humans. Cell based technologies provide a way to model numerous human diseases within a culture dish. To do this, differentiated cells are required and isolation and long-term cultures present potential difficulties. With the use of urine-derived stem cells whether they are iPSCs or not, can be used for modeling multiple different conditions. They can model several metabolic, urinary, nervous, cardiovascular, hemorrhagic, muscular, and autoimmune conditions. Hemophilia has been studied with disease models. IPSCs were generated from human urine-derived stem cells and were then further differentiated into hepatocytes that did not make factor 8, which is a blood coagulation factor that mimics hemophilic patients. Then, with the use of CRISPR-Cas9, it can manipulate the genes to express factor 8 which was done to a mouse in a research study [15].
All this research has just been mainly limited to animal studies and have not been used in human studies because it is still new. It takes a long time for potential treatments to reach human trials and for good reason. There are certain limitations to researching stem cells. These limitations apply to essentially all adult stem cells with the first limitation being that stem cells from somatic adult cells cannot be made into all cell types. They can be made into many different types of cells as shown through the numerous research topics for potential application, but not all cell types. A large area for concern is the formation of tumors and teratomas when implanting stem cells as well as immunological rejection or other adverse effects from stem cell transplants [16]. Moreover, one needs to account for variability. Since all of the applications are newer and many still in the research phase, we do not know everything. Every individual has a distinct genetic background that is prone to various results when conducting research. Finally, an additional limitation of stem cell research is the aging effects on stem cells which is similar to variability. It is known that aging has detrimental effects on stem cells: loss of lineage specificity, loss of self-renewal, cessation of cellular division, and potential malignant transformation [15].
Discussion
Stem cells from human urine can be used for a variety of clinical applications. One clinical application is the ability to obtain renal cells through urine-derived stem cells. Less is known about the cellular growth and in-vitro characteristics of renal cells; however, it is known that they do have they do have less expandability than urine cells. This means that they have a shorter life span than urine-derived stem cells and limited differentiation. This does not mean they are useless. Renal cells derived from human urine were converted into neural stem cells. The renal cells were turned into three different kinds of neural cell types from the induced neural progenitor cells - astrocytes, oligodendrocytes, and neurons. This is a very promising option for treating and potentially curing neurodegenerative diseases. Additionally, this method does not use transcription factors or cause possible modifications to the genome. They can also be used for creating endoderm-derived cells. There is a high expression of Sox-17, so this suggests that it can be used for generating cells for the liver, thyroid, or pancreas [1]. Again, they are the easiest stem cells to obtain and isolate since the cells originate from urine.
There are several other applications for the use of urine-derived stem cells. Organ-specific cells are more ideal for the treatment of the organ they come from. If one has specific cells from urine and renal cells, this will work well for renal reconstruction, kidney bioengineering, and genitourinary repair. Since urine-derived cells can differentiate into myocytes, smooth muscle, endothelial, and epithelial cells; this creates most of the renal tissue which can then be used for renal reconstruction. If one makes a three dimensional arrangement of these cells, it can generate a kidney-like structure that can potentially have effective function. The idea of bio artificial kidneys is gaining more research and clinical application. Endothelial and MSCs have been proven to reduce the development of chronic kidney disease in clinical trials. Additionally, another application of urine-derived stem cells is for genitourinary repair. It is the simplest procedure out of all of the applications because the stem cells do not need to differentiate. It has been shown that urine stem cells are functionally capable to treating end-stage bladder disease and bladder pain syndrome. More studies are being done to utilize urine stem cells for erectile dysfunction as well. Since urine-derived stem cells can differentiate into smooth muscle, this can also show potential for muscle engineering [1].
Moreover, urine-derived stem cells have shown to have the capacity to differentiate into cardiomyocytes. Not much research has been done in this area, but it does have much research potential. Urine-derived stem cells can differentiate into hepatocytes. This is useful for potential stem cell liver transplantation. In instances of acute liver failure, transplantation is the only choice for treatment, and most patient will pass away if they do not get a transplant [1]. Dental tissue can also be created from urine stem cells. One study showed that 30% of transplanted sheets of epithelial cells developed into amelo blasts in a tooth-like structure in mice [1]. Additionally, it can be used for the treatment of diabetes. According to the CDC, 37.3 million people are diagnosed with diabetes in the United States, and this number is growing [17]. Islet transplantation has been executed from cells of cadavers; however, the quality of those cells is not as satisfactory. Furthermore, beta-cell transplantation needs a large quantity of cells in order to be successful. Urine-derived stem cells have the quantity and quality that these transplants require. Renal cells are also able to differentiate into insulin-secreting cells. Multiple studies have been conducted on stem cell use for neuro-regeneration. Though, it can take a long time to generate functional neurons, and the new neural cells can lose some functionality due to the transfer of the cells from the in-vitro adherent culture. There is a different and more efficient approach that can utilize urine stem cells. This method is to generate cells capable of maturation after transplant [1]. Also, urine stem cells can be used for bone engineering. Stem cells are already being used to create bone grafts, but urine-derived stem cells can also be used for the generation of bone grafts as well. There is an ample supply of urine stem cells to use for conditions such as osteoporosis [11].
Recent Research
Moving onto current research, it has been known for a while that stem cells can be found in urine, but what is the latest research discovering? One of latest studies by researchers at the Wake Forest Institute for Regenerative Medicine specifically focuses how the telomerase activity affects the regenerative potential in urine-derived stem cells. A telomere is essentially the cap at the end of the chromatid, and it is a repeating DNA sequence at the end of the body’s chromosome. Telomere length is very important to maintain in order to ensure cell development and survival. If the telomeres become too short, it will activate a DNA damage response that will terminate the cell cycle. To counteract this, stem cells can utilize telomere elongation mechanisms through the use of telomerase. Telomerase is an enzyme that is vital for the self-renewal and differentiation capabilities of stem cells as well as their longevity [18]. This study was conducted to learn about the importance of telomerase activity and looking at surface markers, multipotent differentiation capability, chromosomal stability as well as the potential formation of tumors and teratomas when using urine-derived stem cells. Another aspect from the introduction of this study was that urine-derived stem cells display a lower number of gene markers of stem cells yet provide robust proliferative potential. That is an interesting finding since those normal gene markers allow for proliferation and pluripotency.
Materials and Methods
The researchers collected and cultured urine-derived stem cells from male human urine ages 25-57 years old and divided them into four groups – 20, 30, 40, and 50 years of age. One hundred seventeen urine-derived stem cell clones were isolated, expanded, and categorized. The cells were grown in culture medium comprised of embryonic fibroblast and keratinocyte serum-free medium mixed to a ratio of 1:1. Then, a telomerase activity assay was preformed using the Telo-TAGGG Telomerase PCR-ELISA plus kit. Human bone marrow-derived stem cells and smooth muscle stem cells were used for the negative control, and HEK 293 cells were used forthe positive control. A spectrophotometer was used to measure the absorbance value sat 450nm and read against a blank which was at 690nm. The relative telomerase activity within different samples in the experiment were found using a specific formula. To further establish the effect of time on telomerase activity, the relative telomerase activity of two sets of urine-derived stem cells (ages 20–50 years), positive telomerase activity (TA+) and negative telomerase activity (TA−), were measured after every five passages or at the end passage.
The urine-derived stem cells were seeded in a well plate for cell proliferation to be assessed. They were plated in 96-well plates, and the culture medium was changed every second day. Cell proliferation was assessed on days 1, 3, 5, and 7 by using an MTS cell proliferation assay kit. The MTS reagent was incubated, and afterwards, the absorbance was measured by a spectrophotometer at 490nm. The doubling time and population doubling calculations were found based on single urine-derived stem cells at passage zero up to passage 16 for each clone. Flow cytometry was used to assess the cell surface markers of urine-derived stem cell clones. The analysis stained the urine-derived stem cells with explicit labeled anti-human antibodies: CD25-PE, CD31-FITC, CD34-FITC, CD44-FITC, CD45-FITC, CD73-PE, CD90-FITC, CD105-PerCP-CY5.5, CD117-PE, CD140b-PE, and CD146-PE. They incubated urine-derived stem cells on ice and used conjugated iso-type control antibodies to establish background fluorescence. Then, the cells were washed and analyzed with flow cytometry.
The capacity to differentiate was analyzed and their changes in morphology and histochemical staining for certain components were logged. The smooth muscle cell induction, uroepithelial induction, and osteogenic induction were tested. The researchers performed western blotting on the proteins of smooth muscle cell and urothelial cell induced to urine-derived stem cells that had both positive and negative telomerase activity. In order to evaluate the barrier function of these induced cells, the researchers performed a permeability assay, and the results were plotted as a percentage that was relative to the control, which was no cell. Additionally, they implemented a karyotype analysis to assess the chromosomal stability of cultured urine-derived stem cells in early passage and late passage. A standard cytogenetic analysis was completed under microscopy, then chromosomal image capture and karyo-typing were executed using CytoVision.
Finally, the researchers executed a soft agar assay in vitro and a spontaneous transformation in vivo assay for analyzation of tumor and teratoma growths in nude mice. The agar assay was used to differentiate tumor cells from non-transformed or normal cells because normal cells do not undergo anchorage-independent growth or flourish on an agar medium. The spontaneous transformation in vivo assay was used to determine the non-tumorigenicity of urine-derived stem cells with positive telomerase activity in vivo. A human ESC line was used for the positive control. Eight weeks after the mice were injected with the cells, they were sacrificed, and their kidneys were evaluated. For the assessment of teratoma formation and general graft histology, H&E staining was performed [19].
Summary & Conclusions
The first part of the experiment was analyzing the telomerase activity in urine-derived stem cells based on the age of the donor. A total of 80 urine stem cells clones from ten individuals should a detectable amount of telomerase activity. The highest amount of telomerase activity was from the donors in their 20’s and it decreased very slightly the older the donors got with the largest decrease in activity in the donors in their 50’s as seen in (Figure 1).
Figure 1: Distribution of human USCsTA+ in different age groups. Most USCs (76.9–72.7%) retained telomerase activity in donors at age 20–40 years of age, but the ratio of USCsTA+ significantly dropped to 59.2% in the donors over 50 years [19].
Going back to research limitations that were discussed earlier in the article, age plays a role in the telomerase activity as well and needs to be considered when going forward with future clinical applications. (Table 1) gives more of the numerical values seen in (Figure 1).
Table 1: The rate, mean, and highest telomerase activity of the isolated USCsTA+ clones for individual donors (p2) in the four different age groups [19].
Another result was focused on if the telomerase activity in human urine stem cells decreased as the number of passages progressed. They measured two pairs of urine-derived stem cells that were positive and negative telomerase activity at early and late passage. (Figure 2) shows that even if there is high amount of telomerase activity to begin with, the activity and strength of telomerase decreased within the clones in the passages during the culture in vitro. Therefore, a TA+ clone that has been through multiple passages can be seen as practically equivalent to a TA- clone.
Figure 2: The relative telomerase activity of USCTA+ clones declined gradually in the passages during culture in vitro [19].
The next results are looking at the cell renewal ability of urine-derived stem cells. The negative and positive telomerase activity clones from a single donor were tested as shown in Figure [3]. Urine stem cells that are telomerase activity positive generated more cells and took longer to reach cell senescence. This is when the cells stop dividing and there are noticeable phenotypic alterations. The positive telomerase activity clone maintained a “rice-grain” appearance all the way to passage 16. The negative telomerase activity clone had a flattened cell shape and steadily displayed a larger appearance. Negative telomerase activity reacted senescence at passage 9, and positive telomerase activity reacted senescence at passage 16.
Figure 3: Changes in the morphologic appearance of USCsTA+ and USCsTA− at different passages [19].
(Figure 4) is a cell growth curve. It shows that positive telomerase activity urine-derived stem cells proliferated more rapidly than the negative telomerase activity cells did. Six individual positive telomerase activity urine stem cell clones (n = 6, p3, A35, B36, G1, E37, J29, and K5) generated significantly more cells and grew faster than negative telomerase activity urine stem cell clones (n = 6, p3, A42, B31, G12, E34, J18, and K6).
Figure 4: Cell growth curve of USCsTA+ vs. USCsTA−. Cell proliferation was measured as the USCs were cultured for 7 days [19].
Subsequently, the mean doubling times of the positive telomerase activity clones were much shorter than the negative telomerase activity clones. The population doubling of the positive telomerase activity clones also increased significantly when compared to the negative telomerase clones as seen in Table 2. Additionally, the table is comparing the ages of the stem cell clones, and the younger donor cells had a higher population doubling and a shorter doubling time.
Table 2: Population doubling and doubling time of USCTA+ vs USCTA−in the 20 and 50 years age groups in early and late passages to determine if there was a difference in telomerase activity with increasing age [19].
(Figure 5) has multiple aspects. Graph A shows that positive telomerase activity has a higher population doubling as well as graph B shows that it has a quicker doubling time. Graph C demonstrates that positive telomerase activity has rapid proliferation, generates more cells, and the cells survive longer than negative telomerase activity cells.
Figure 5: Population doubling, doubling time, and in vitro survival time course of USCsTA+ vs USCsTA−[19].
Additionally, this study found that regardless of telomerase activity, the clones had similar surface markers. This is interesting in general since urine-derived stem cells have overall fewer surface markers than other stem cells. Positive telomerase activity urine stem cell clones (A35, B36, G1, E37, J29, and K5) and negative telomerase activity urine stem cell clones (A42, B31, G12, E34, J18, and K6) exhibited sets of MSC surface markers (CD44, CD90, CD73, CD105, and CD146), positive at comparable levels, while both positive and negative telomerase activity clones did not show markers of haemopoietic stem cells (i.e., CD31, CD34, CD45, CD25, and CD117). Yet, three out of five positive telomerase activity clones displayed the CD105 surface marker, but there was no or weak expression in the four negative telomerase activity clones cell clones. MSC surface markers of urine-derived stem cells at passage 4 were identified by flow cytometry as shown in (Figure 6).
Figure 6: USCs expressing MSC surface markers [19].
(Figure 7) displays the results for the potent differentiation capacity of the positive telomerase activity cells. Figure A was induced to the urothelial lineage (cells from the lining of the urinary tract). Figure B shows the induced to the smooth muscle lineage. Finally, Figure C shows that the telomerase positive cell could be induced into osteocytes. These results show that positive telomerase activity cells performed better in terms of multiple differentiation capacity in osteogenic, myogenic, and uroepithelial differentiation than negative telomerase activity cells, indicating that telomerase is required for not only cell proliferation but also multiple differentiation in human urine derived stem cells.
Figure 7: USCs undergo multi-potential differentiation in vitro [19].
The next result of this study was that the karyotype remained stable in the urine-derived stem cells as shown in Figure 8. Figure A shows that regardless of telomerase activity the cells displayed a normal karyotype and that there was no multiploidy or chromosomal rearrangements in metaphase. Figure B displays that telomerase positive clones continuedto stay the same size on day 1 through 14. Though, the cancer cell in the first column did form large colonies. Lastly, Figure C shows that no teratomas were found when the telomerase positive clone A35 was implanted into the kidney of mice after 8 weeks. Additionally, all human H9 lines produced three embryonic germ layers.
Figure 8: Spontaneous transformation assays of USCTA+ clones [19].
In conclusion, (Table 3) shows the summary findings of this study. Again, there was a higher population doubling and shorter doubling time in positive telomerase activity cells. They have strong positive markers for cell differentiation. Both degrees of telomerase activity were the same for MSC markers expect for CD105. The 4 out of 6 telomerase positive cells expressed CD105 and only 2 out 6 of the telomerase negative cells expressed it. The urine-derived stem cells do better overall if they are from younger donors, but they are even stronger if they have positive telomerase activity. Finally, regardless of telomerase activity they both have chromosome stability and do not form tumors or teratomas in vivo. These are very promising and interesting outcomes from this study for further research and clinical application. This study indicates that that urine-derived stem cells with positive telomerase activity act like a subpopulation with potential regeneration capacity in regard to cell proliferation and multiple differentiation.
Table 3: Feature summary of human USCs with telomerase activity in USCTA+ and USCTA−clones [19].
This study is a positive step forward for the evaluation of urine-derived stem cells. If research continues to progress in regard to urine-derived stem cells, it will be a viable source for regenerative medicine. It does not require an invasive procedure to harvest the stem cells, and there is an endless supply since the stem cells originate from urine samples. The use of each individual’s autologous stem cells elevates the possibility for immunological rejection or other negative immune side-effects. Nevertheless, much more extensive investigation is needed before they can be used for clinical application. Referring back to the limitations of research with urine stem cells, every individual has a diverse genetic background. In both urine derived stem cells and iPSCs, the variability of the genomes can potentially obscure the data and make it more difficult to reproduce the experiment. Additionally, it may be an issue to ensure sterile and clinical-grade urine-derived stem cells. Although the method for sterilization is fairly accurate, it is vital to guarantee the stem cells are not contaminated for clinical use. A third caveat involves issues with reprogrammed iPSCs. Re-programing somatic cells leaves room for variation in epigenetic memory and differentiation potentials [20]. It is important to further study and note these complications in urine stem cell research. Future studies should analyze physiological elements that regulate the patterns of positive telomerase urine stem cells as well as the physiological factors that impact the cells’ ability to partake in cell therapy. It would be beneficial to study how telomerase activity effects the paracrine mechanisms of urine derived stem cells. Additionally, it would be useful to examine the variations in the cell subpopulation such as the effects of aging [19].
References
- Pavathuparambil Abdul Manaph N, Al-Hawwas M, Bobrovskaya L, Coates PT, Zhou XF. 2018 Urine-derived cells for human cell therapy - stem cell research & therapy. Stem Cell Res Ther. 9(1):189.
- https://www.healthline.com/health/is-urine-sterile.
- Zhou Q, Cheng Y, Sun F, Shen J, Nasser M I, et al. (2022) A comprehensive review of the therapeutic value of urine-derived stem cells. Front Genet. 12:781597.
- https://www.mayoclinic.org/tests-procedures/bone-marrow-transplant/in-depth/stem-cells/art-20048117
- https://www.niddk.nih.gov/health-information/urologic-diseases/hydronephrosis-newborns/vesicoureteral-reflux
- Zhang Y, McNeill E, Tian H, Soker S, Andersson KE, et al. (2008) Urine derived cells are a potential source for urological tissue reconstruction. J Urol. 180(5):2226:33.
- Bharadwaj S, Liu G, Shi Y, Wu R, Yang B, et al. (2013) Multipotential differentiation of human urine-derived stem cells: Potential for therapeutic applications in urology. Stem Cells. 31(9):1840-56.
- https://www.mayoclinic.org/diseases-conditions/diabetic-nephropathy/symptoms-causes/syc-20354556
- Jiang Z zhen, Liu Y mei, Niu X, Yin Jyong, Hu B, et al. Exosomes secreted by human urine-derived stem cells could prevent kidney complications from type I diabetes in rats - stem cell research & therapy. Stem Cell Res Ther. 7:24.
- Wang L, Chen Y, Guan C, Zhao Z, Li Q, et al. (2017) Using low-risk factors to generate non-integrated human induced pluripotent stem cells from urine-derived cells. Stem Cell Res Ther. 8(1):2017.
- Chen CY, Rao SS, Ren L, Hu XK, Tan YJ,et al. (2018)) Exosomal DMBT1 from human urine-derived stem cells facilitates diabetic wound repair by promoting angiogenesis. Theranostics. 8(6):1607-1623.
- Cao Y, Xu J, Wen J, Ma X, Liu F, et al. (2018) Generation of a urine-derived IPS cell line from a patient with a ventricular septal defect and heart failure and the robust differentiation of these cells to cardiomyocytes via small molecules. Cellular Physiol Biochem. 50(2):538-51.
- Chen CY, Rao SS, Tan YJ, Luo MJ, Hu XK, et al. (2019) Extracellular vesicles from human urine-derived stem cells prevent osteoporosis by transferring CTHRC1 and OPG. Bone Res. 7:18.
- Bento G, Shafigullina AK, Rizvanov AA, Sardão VA, Macedo MP, et al. (2020) Urine-derived stem cells: Applications in regenerative and Predictive Medicine. Cells. 9(3):573.
- Ikehara S. (2010) Grand challenges in stem cell treatments. Front Cell Dev Biol. 1:2.
- https://www.cdc.gov/diabetes/data/statistics-report/index.html
- Li JSZ, Denchi EL. (2018) How stem cells keep telomeres in check. Differentiation. 100:21-25.
- Shi Y, Liu G, Wu R, Mack DL, Sun XS, et al. ( 2022) Differentiation capacity of human urine-derived stem cells to retain telomerase activity. Front Cell Dev Biol. 10:890574.
- Ji X, Wang M, Chen F, Zhou J. (2017) Urine-derived stem cells: The present and the future. Stem Cells int. 2017:4378947