Regenerative Medicine in Spine Care: Clinical Potential of Mesenchymal Stem Cells for Chronic Low Back Pain
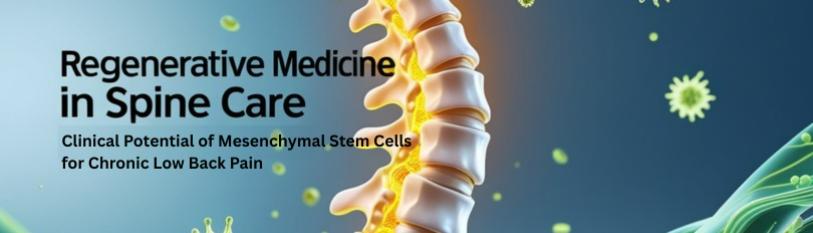
Catherine Capparelli and Vincent S. Gallicchio*
Department of Biological Sciences College of Science Clemson University Clemson, SC 29634
*Corresponding author: Vincent S. Gallicchio, Department of Biological Sciences 122 Long Hall College of Science Clemson University 297 Parkway Drive Clemson, SC 29634
Citation: Capparelli C and Gallicchio VS, Regenerative Medicine in Spine Care: Clinical Potential of Mesenchymal Stem Cells for Chronic Low Back Pain. J Stem Cell Res. 6(1):1-19.
Received: April 25, 2025 | Published: May 05, 2025
Copyright© 2025 genesis pub by Capparelli C et al. CC BY-NC-ND 4.0 DEED. This is an open-access article distributed under the terms of the Creative Commons Attribution-NonCommercial-No Derivatives 4.0 International License.,This allows others to distribute, remix, tweak, and build upon the work, even commercially, as long as they credit the authors for the original creation.
DOI: https://doi.org/10.52793/JSCR.2025.6(1)-72
Abstract
Chronic low back pain (CLBP) remains one of the leading causes of disability worldwide, with current treatments often providing only symptomatic relief. Among emerging regenerative strategies, mesenchymal stem cell (MSC) therapy has garnered significant attention for its ability to address the underlying pathophysiology of CLBP, particularly intervertebral disc degeneration (IDD). This paper reviews the therapeutic mechanisms of MSCs, including their capacity for disc regeneration, immunomodulation, and pain alleviation via paracrine signaling and anti-inflammatory effects. Preclinical studies demonstrate that MSCs restore disc structure, inhibit neuroinflammation, and enhance extracellular matrix composition. Human clinical trials further indicate that both autologous and allogeneic MSCs can lead to significant reductions in pain and functional disability, with minimal adverse effects. While promising, MSC therapy faces challenges related to variability in cell sources, delivery methods, regulatory frameworks, and long-term outcome data. Comparisons with other regenerative approaches—such as platelet-rich plasma (PRP), gene therapy, and tissue engineering—highlight MSCs’ unique advantages in cellular replacement and sustained modulation of the disc environment. Future directions emphasize the integration of MSCs with biomaterials and PRP in combination therapies, as well as the need for long-term, large-scale clinical trials to validate efficacy, optimize protocols, and ensure safety. With continued interdisciplinary research and technological innovation, MSC therapy holds substantial potential to revolutionize the treatment paradigm for CLBP by shifting from palliative care to regenerative repair.
Keywords
Mesenchymal Stem Cells (MSCs); Chronic Low Back Pain (CLBP); Intervertebral Disc Degeneration (IDD); Regenerative Medicine, Cell Therapy.
Abbreviations
AD-MSCs – Adipose-Derived Mesenchymal Stem Cells
AF – Annulus Fibrosus
ATF3 – Activation Transcription Factor 3
Bcl-2 – B-cell Lymphoma 2
BM-MSCs – Bone Marrow-Derived Mesenchymal Stem Cells
BMC – Bone Marrow Concentrate
CEPs – Cartilaginous Endplates
CLBP – Chronic Low Back Pain
CRISPR-Cas9 – Clustered Regularly Interspaced Short Palindromic Repeats–Associated Protein 9
DHI – Disc Height Index
DLBP – Discogenic Low Back Pain
ECM – Extracellular Matrix
ESIs – Epidural Steroid Injections
EVs – Extracellular Vesicles
GMP – Good Manufacturing Practice
GMH – Global Mental Health
GPH – Global Physical Health
HA – Hyaluronic Acid
HCT/Ps – Human Cells, Tissues, and Cellular and Tissue-Based Products
HSCs – Hematopoietic Stem Cells
HUCMSCs – Human Umbilical Cord Mesenchymal Stem Cells
IDD – Intervertebral Disc Degeneration
IDP – Intervertebral Disc
IGF-1 – Insulin-Like Growth Factor-1
IL-1β – Interleukin-1 Beta
IL-6 – Interleukin-6
IL-10 – Interleukin-10
Iba-1 – Ionized Calcium-Binding Adapter Molecule 1
IVD – Intervertebral Disc
LFJA – Lumbar Facet Joint Arthropathy
mTOR – Mammalian Target of Rapamycin
MMPs – Matrix Metalloproteinases
MRI – Magnetic Resonance Imaging
MSC(s) – Mesenchymal Stem Cell(s)
NRS – Numeric Rating Scale
NP – Nucleus Pulposus
NSAIDs – Nonsteroidal Anti-Inflammatory Drugs
ODI – Oswestry Disability Index
PDGF – Platelet-Derived Growth Factor
PRP – Platelet-Rich Plasma
PROMIS CATs – Patient-Reported Outcomes Measurement Information System Computer Adaptive Tests
SOX9 – SRY-Box Transcription Factor 9
TGF-β – Transforming Growth Factor Beta
TIMP-1 – Tissue Inhibitor of Metalloproteinases-1
TNF-α – Tumor Necrosis Factor-Alpha
UC-MSCs – Umbilical Cord-Derived Mesenchymal Stem Cells
VAS – Visual Analog Scale
VEGF – Vascular Endothelial Growth Factor
Introduction
Background on Chronic Back Pain
Chronic low back pain (CLBP), defined as low back pain that persists for more than three months, is one of the most prevalent and disabling musculoskeletal disorders worldwide. Estimates suggest that nearly 577 million people are affected by low back pain globally, with CLBP comprising a significant proportion of these cases [1]. Lifetime prevalence is strikingly high, with up to 80% of individuals experiencing at least one episode of low back pain, and between 5% and 25% of these progressing to chronicity [2,3].
CLBP case frequency increases steadily with age, peaking between ages 30 and 60, and is more commonly reported amongst women [1,2]. The persistent pain significantly interferes with physical functioning, sleep, and overall well-being. Even in the absence of identifiable structural pathology–as is the case in 90-95% of low back pain diagnoses classified as “nonspecific” –CLBP has been strongly associated with impaired quality of life across physical, emotional, and social domains [3]. Despite the high global prevalence of CLBP, its management remains a clinical challenge, often characterized by limited long-term efficacy across treatment modalities. Approaches to CLBP usually encompass conservative management, interventional procedures, and surgical repairs.
Conservative management–including physical therapy, exercise, and patient education–remains the recommended first-line approach for CLBP. International clinical guidelines promote early use of non-pharmacological treatments such as structured exercise programs, cognitive-behavioral strategies, and physical activity over passive modalities like rest or medication. However, no single exercise modality has demonstrated clear superiority, and access to consistent, structured programs remains uneven across care settings [4]. Moreover, although psychoeducation and integrative behavioral therapies can improve functional outcomes and quality of life, these approaches require sustained patient engagement and interdisciplinary support that may not be readily available in all primary care contexts [5].
Interventional treatments such as epidural steroid injections (ESIs) are frequently used for radicular or neuropathic components of CLBP. While ESIs may provide short-term relief in some patients, particularly those with lumbosacral radiculopathy or disk herniation, their long-term efficacy remains inconsistent. Meta-analyses have found that outcomes such as pain reduction and functional improvement are not significantly superior to placebo or anesthetic-only injections, casting doubt on the widespread use of ESIs in chronic cases [6,7]. In fact, current guidelines increasingly advise caution or even recommend against the routine use of ESIs for CLBP, citing insufficient evidence for sustained benefit [8].
Surgical intervention, including decompression and spinal fusion, is typically reserved for cases refractory to conservative and interventional treatments or for patients with specific pathologies such as spinal instability or severe disk herniation. However, the outcomes of surgical procedures for non-specific CLBP remain mixed. Postoperative improvements in pain and function are not guaranteed, and surgery carries inherent risks including infection and prolonged recovery periods [5,8]. As such, surgical treatment is usually a last resort.
Introduction to Mesenchymal Stem Cells (MSCs)
Mesenchymal stem cells (MSCs) are multipotent stromal cells capable of self-renewal and differentiation into various mesodermal lineages, including osteoblasts, chondrocytes, and adipocytes [9]. First characterized in the 1970s by Friedenstein et al., MSCs were initially isolated from bone marrow and identified by their plastic adherence and colony-forming capacity. Since then, they have been found in various adult and perinatal tissues such as adipose tissue, umbilical cord, dental pulp, placenta, and peripheral blood [10,11].
Figure 1: MSCs are isolated from several sources and can differentiate into different types of cells [59].
The biological activity of MSCs is predominantly mediated by their paracrine effects, which include secretion of growth factors, cytokines, and extracellular vesicles (EVs) such as exosomes. These factors contribute to angiogenesis, immunomodulation, anti-apoptosis, and matrix remodeling, making MSCs powerful agents in tissue regeneration and inflammation regulation [12,13]. MSCs modulate immune responses through mechanisms involving surface molecules (e.g., HLA-G) and soluble factors like transforming growth factor-β (TGF-β), and interleukin-10 (IL-10), enabling them to suppress both innate and adaptive immune activity when overactivated [12].
Several tissues serve as viable sources for MSC isolation, with each exhibiting distinct proliferative capacity, immunomodulatory profiles, and differentiation potential. Bone marrow-derived MSCs (BM-MSCs) are the most extensively studied and remain a gold standard in orthopedic and spinal regenerative applications due to their robust osteogenic potential [9,10]. Adipose-derived MSCs (AD-MSCs), harvested through minimally invasive liposuction procedures, are noted for their higher yield and proliferative advantage, making them appealing for clinical applications requiring large cell numbers [11]. Umbilical cord-derived MSCs (UC-MSCs), collected from perinatal tissue, offer immunologic naivety, higher proliferation rates, and lower ethical barriers, suggesting promise in allogeneic transplantation settings [10,13].
Purpose of the study
Given the limitations of current conservative, interventional, and surgical treatments for chronic low back pain (CLBP), there has been growing interest in regenerative medicine approaches that aim not merely to alleviate symptomatic pain but to restore damaged intervertebral disc tissue. Among these, MSCs have emerged as promising therapeutic agents due to their multipotency, paracrine signaling properties, and capacity to modulate the immune response and microenvironment of degenerated discs [14].
Preclinical and early-phase clinical studies have demonstrated that MSC therapy can reduce pain, improve disc hydration, and enhance functional outcomes in patients with discogenic low back pain [14,15]. However, results across trials remain heterogeneous, with variability in MSC sources, delivery techniques, dosing regimens, and patient selection. Despite promising findings—including significant reductions in visual analog scale (VAS) and Oswestry Disability Index (ODI) scores in randomized controlled trials—the long-term efficacy and optimal therapeutic parameters of MSC-based therapies are still under investigation [15].
Nevertheless, translational barriers such as inconsistent manufacturing protocols, lack of standardized outcome measures, and incomplete understanding of the discogenic microenvironment continue to limit widespread clinical implementation [16,17]. This study aims to explore the therapeutic potential of MSC-based interventions for chronic back pain, particularly in the context of intervertebral disc degeneration. By reviewing and synthesizing current evidence on MSC biology, mechanisms of action, and clinical outcomes, the aim is to clarify their role in regenerative therapy and identify the critical challenges that must be addressed to optimize future clinical applications.
Pathophysiology of chronic back pain
Chronic low back pain (CLBP) is frequently attributed to intervertebral disc degeneration (IDD), a multifactorial and progressive condition that disrupts the structural, cellular, and biochemical integrity of the intervertebral disc (IVD). The IVD comprises three key components: the central nucleus pulposus (NP), the annulus fibrosus (AF), and the cartilaginous endplates (CEPs). Under normal conditions, the disc matrix is rich in proteoglycans and water, maintaining osmotic pressure necessary for load absorption and spinal flexibility [18].
The degenerative cascade begins with an imbalance between catabolic and anabolic processes in the NP, often due to aging, genetic predisposition, and environmental stressors such as repetitive loading or hypoxia [19,20]. This results in a progressive loss of proteoglycans and water content, causing structural weakening, disc desiccation, and height reduction. As the AF undergoes fibrotic changes, microfissures form, permitting ingrowth of nociceptive nerve fibers and neovascularization, processes absent in healthy discs [18,21]. These changes render the IVD not only structurally compromised but also pain-sensitive, contributing to discogenic pain.
Recent studies highlight the inflammatory microenvironment as a key driver of disc degeneration and pain. Elevated levels of pro-inflammatory cytokines, including interleukin-1β (IL-1β), tumor necrosis factor-alpha (TNF-α), and IL-6, are consistently found in degenerated IVDs. These cytokines promote matrix degradation via upregulation of matrix metalloproteinases (MMPs) and ADAMTS proteases, leading to further loss of extracellular matrix (ECM) integrity [18,22]. Simultaneously, these cytokines sensitize sensory neurons and promote the expression of pain mediators such as prostaglandin E2, nitric oxide, and nerve growth factor, thereby amplifying nociceptive signaling [18,21].
Moreover, degeneration-induced breach of immune privilege in the NP exposes previously sequestered antigens to the immune system, inciting autoimmune-like responses that recruit immune cells such as macrophages and T lymphocytes [21]. This infiltration not only exacerbates inflammation but also contributes to sustained pain, even in the absence of overt mechanical compression.
Importantly, IDD-associated structural alterations affect adjacent spinal elements, including facet joints, ligaments, and paraspinal musculature, potentially causing spinal instability and further biomechanical stress. These secondary effects may create a feedback loop that perpetuates degeneration and chronic pain [20,22].
Taken together, the pathophysiology of CLBP extends beyond mechanical degeneration to encompass complex biochemical, immunological, and neurovascular factors, suggesting that effective treatment must address not only structural deterioration but also the underlying inflammatory and neuropathic components of pain.
Figure 2: Pathophysiological model of chronic low back pain [58].
Therapeutic Mechanisms of MSCs
Mesenchymal stem cells (MSCs) exert a multifaceted therapeutic role in the treatment of chronic back pain, particularly in cases associated with intervertebral disc degeneration (IDD). Their effectiveness derives from three principal mechanisms: regeneration of disc tissue, modulation of the inflammatory microenvironment, and alleviation of pain via neuromodulatory effects.
Regenerative potential
MSCs contribute to the regeneration of degenerated intervertebral disc (IVD) tissue by differentiating into nucleus pulposus (NP)-like cells and promoting extracellular matrix (ECM) production. These cells can restore the balance between anabolic and catabolic processes within the disc by synthesizing type II collagen, aggrecan, and other proteoglycans essential for disc hydration and resilience [23]. Furthermore, MSCs stimulate endogenous repair by enhancing the proliferation and matrix synthesis of resident disc cells through the secretion of trophic factors [24]. This process is supported by paracrine signaling mechanisms, often referred to as the MSC secretome, which includes growth factors such as transforming growth factor-β (TGF-β), vascular endothelial growth factor (VEGF), and insulin-like growth factor-1 (IGF-1) [25].
Anti-inflammatory effects
A critical feature of MSCs is their robust immunomodulatory capability. MSCs reduce the expression of key pro-inflammatory cytokines implicated in IDD progression, including tumor necrosis factor-α (TNF-α), interleukin-1β (IL-1β), and IL-6 [23,24]. These actions mitigate matrix degradation and apoptosis within the NP, helping to preserve disc structure and function. Additionally, MSC-derived extracellular vesicles (EVs), including exosomes, have been shown to carry anti-inflammatory miRNAs and proteins that further support tissue repair while avoiding immune rejection [26]. These vesicles are now recognized as major effectors in MSC-mediated disc regeneration due to their ability to cross biological barriers and act at distant sites.
Figure 3: The multi-faceted anti-inflammatory actions of MSCs. In response to pro-inflammatory cytokines or TLR3 stimuli, MSCs will develop an anti-inflammatory profile. Through the secretion of soluble factors these licensed cells can act on numerous innate immune cells affecting both effector function and phenotype [60].
Pain modulation
Beyond structural repair, MSCs play a pivotal role in the modulation of pain. Preclinical studies have demonstrated that MSC administration reduces mechanical allodynia and hyperalgesia in models of neuropathic and discogenic pain [27,28]. This antinociceptive effect is achieved by inhibiting neuroinflammation, promoting remyelination, and downregulating markers of neural injury such as ionized calcium-binding adapter molecule 1 (Iba-1) and activation transcription factor 3 (ATF3) in dorsal root ganglia [27]. Additionally, MSCs interfere with pain signaling pathways by reducing the production of pain mediators like prostaglandin E2, nitric oxide, and nerve growth factor within degenerated discs [23].
Collectively, MSCs present a compelling therapeutic avenue for treating chronic back pain by addressing its underlying degenerative, inflammatory, and neuropathic components. Ongoing research seeks to refine delivery strategies, optimize cell sourcing, and leverage acellular approaches such as exosome therapy to enhance clinical outcomes.
Clinical Evidence and Studies on MSC Therapy for CLBP
Given the complex pathophysiology of chronic low back pain (CLBP) and the multifaceted therapeutic mechanisms of mesenchymal stem cells (MSCs), it becomes essential to evaluate how these biological capabilities translate into real-world therapeutic outcomes. Both preclinical animal studies and early-phase clinical trials have been instrumental in validating the regenerative, anti-inflammatory, and analgesic potential of MSCs.
Preclinical studies and animal models
A systematic review and meta-analysis evaluated 34 randomized controlled trials assessing the efficacy of stem cell therapy in animal models of intervertebral disc degeneration (IDD), a key contributor to chronic low back pain. The analysis encompassed over 1,100 discs across four species—rabbits, rats, mice, and sheep—and assessed imaging-based outcomes such as disc height index (DHI), T2-weighted MRI signal intensity, and MRI grading scores. Stem cell therapy significantly improved DHI and MRI parameters in rabbits (P < 0.001), mice (P < 0.001), and rats (P = 0.001), suggesting that MSCs can restore disc hydration and structure. However, outcomes in sheep were less consistent, indicating potential species-specific responses [29].
Table 1: Forest plot showing the effect of stem cell group and control group on disc height in animal models [29].
Another systematic review focused on mesenchymal stem cell-derived exosomes in bone regeneration, analyzing 23 preclinical studies involving rodent and rabbit models of critical-sized bone defects. Exosome-treated groups consistently exhibited enhanced bone formation, angiogenesis, and biomechanical integrity. These improvements were attributed to paracrine signaling mechanisms, which activated osteogenic pathways, promoted vascularization, and supported cell proliferation and migration. While these studies primarily targeted bone repair, the mechanisms involved are directly relevant to intervertebral disc regeneration due to shared microenvironmental challenges and tissue remodeling processes [30].
In the context of cartilage regeneration, a separate review evaluated 13 animal studies investigating MSC-derived exosomes in models of osteoarthritis and osteochondral injury. Intra-articular delivery of exosomes led to significant improvements in histological scores, matrix deposition, and chondrocyte proliferation. These findings suggest that MSC exosomes can enhance tissue repair without the need for direct cell transplantation, offering a promising, scalable alternative for treating degenerative spinal conditions that involve cartilaginous disc components [31].
Preclinical studies have also examined MSCs and biomaterial scaffolds in spinal cord injury models. Rodent and non-human primate studies revealed that these combinatorial approaches facilitate axonal regeneration, reduce glial scar formation, and support functional motor recovery. While primarily addressing traumatic spinal injury, these findings highlight MSCs' broader neuroregenerative and anti-inflammatory potential—properties relevant for CLBP with neuropathic components [32].
Lastly, a review of MSC-based therapies for cartilage disorders highlighted critical translational challenges observed in animal models, including variability in cell dose, immune response, and the inflammatory microenvironment. While most studies reported symptomatic and structural improvements, heterogeneity in outcome measures underscores the need for standardized protocols before widespread clinical application [33].
Human clinical trials and case studies
A phase II randomized, double-blind, placebo-controlled trial was designed to evaluate the efficacy and safety of autologous adipose-derived mesenchymal stem cells (ADMSCs) combined with hyaluronic acid (HA) hydrogel in the treatment of discogenic low back pain (DLBP). This single-center study enrolled 100 participants with confirmed single-level disc degeneration. Patients were randomized into three treatment arms (low, medium, and high doses of ADMSCs) and one placebo control group in a 1:1:1:1 ratio. All participants underwent liposuction for ADMSC isolation, with transplantation following three weeks of cell expansion. The primary outcome was reduction in pain severity measured by the Visual Analogue Scale (VAS). Secondary endpoints included Oswestry Disability Index (ODI), Japanese Orthopaedic Association Scores, MRI-based disc morphology (Pfirrmann grade), and safety evaluations such as blood work and immunological assays. Follow-up was scheduled at multiple intervals up to 24 months. This trial is noteworthy for integrating a scaffold (HA hydrogel) with cell therapy and assessing both morphological and symptomatic improvement in a rigorous controlled setting [34].
A single-center feasibility study assessed the use of low-dose intradiscal ADMSC injections in nine patients with chronic lumbar discogenic pain unresponsive to conservative treatment. Each patient received a single injection of 10 million autologous ADMSCs into the affected disc, with an optional second injection at 6 months. Safety and efficacy were monitored via patient-reported outcomes (VAS, ODI, EQ-5D), MRI, and clinical exams over a 12-month period. At the one-year follow-up, seven patients (78%) reported reduced pain, five (56%) reported improved work capacity, and three (33%) reduced their reliance on analgesics. MRI results indicated stabilization or improvement in disc morphology, including reduced annular fissures and disc protrusions. No serious or unexpected adverse events occurred. These findings support the safety and potential therapeutic benefit of ADMSCs even at low doses, with structural and quality-of-life improvements observed in a difficult-to-treat population [35].
A 12-month open-label, prospective, controlled study investigated the effects of autologous bone marrow-derived mesenchymal stem cells (BM-MSCs) in patients with severe lumbar spinal degeneration involving multiple spinal structures. The treatment group received bone marrow concentrate (BMC) injections simultaneously into the intervertebral discs, facet joints, sacroiliac joints, and perineural regions, while the control group received conventional therapies (NSAIDs, physical therapy, opioids, and spinal injections). Primary outcomes included the Oswestry Disability Index (ODI) and Numeric Rating Scale (NRS-11) for pain, while secondary measures included EQ-5D-3L, Global Mental Health (GMH), and Global Physical Health (GPH). At 12 months, 67% of the treated group achieved a clinically meaningful improvement in ODI, and 56% experienced at least a 2-point reduction in NRS pain scores, compared to 8% and 10% respectively in the control group. Additionally, opioid use decreased in the intervention group but increased in controls. These results demonstrate not only significant symptom relief but also functional recovery following MSC-based regenerative treatment, even in the context of complex, multistructural degeneration [36].
In a case report involving cervical spine compression, a patient received a multimodal MSC-based intervention consisting of adipose-derived MSCs, MSC-derived exosomes, and platelet-rich plasma (PRP) delivered over five days. The protocol included localized infiltrations, intravenous nutritional support, and oxygen therapy. Initial MRI revealed spinal stenosis with mild cord compression at C5–C6. Within three days, the patient reported improved posture, pain reduction, and enhanced balance. At the five-month follow-up, MRI confirmed decreased compression and structural improvement at the affected spinal level. Muscle cramps and spasms were also reduced, indicating neurological and musculoskeletal benefits. While limited by its uncontrolled, anecdotal nature, this case illustrates the rapid onset and multi-system benefits potentially achievable through MSC-based interventions, particularly when integrated into a multidisciplinary care plan [37].
A phase I clinical trial (CellKine study) explored the safety and feasibility of allogeneic BM-MSCs administered intra-articularly for patients with lumbar facet joint arthropathy (LFJA). In this initial report, a patient with chronic facetogenic low back pain unresponsive to corticosteroids and medial branch blocks received 20 million BM-MSCs into bilateral L4–L5 facet joints. Follow-ups over two years assessed pain (VAS), function (Oswestry Disability Index, PROMIS CATs), work status, and MRI changes. The patient showed substantial and sustained improvements in pain, physical function, and mental health. MRI imaging is redundant. MRI revealed in the treated joints, supporting the anti-inflammatory and regenerative effects of MSC therapy. No adverse events were observed, and the findings reinforce the safety profile and long-term benefits of MSCs in facet-mediated low back pain [38].
Figure 4: Sagittal and axial Dixon MRI images of the right L4–L5 facet joint taken before (A, B) and 12 months after (C, D) bone marrow-derived mesenchymal stem cell (BM-MSC) injection. Pre-injection images (A and B) show increased signal intensity (indicative of edema) in the posterior facet joint capsules (highlighted by yellow arrows), with lateral spread into the right L4–L5 neural foramen and edema in the right ligamentum flavum—findings consistent with grade 4 facet synovitis. Post-injection images (C and D) show decreased edema within the facet joint capsule. A small residual abnormal signal remains at the posterior margin of the synovial capsule, consistent with grade 1 facet synovitis [38].
Comparison with other regenerative approaches
As mesenchymal stem cell (MSC) therapies gain traction as regenerative treatments for chronic low back pain (CLBP), it is crucial to contextualize their potential alongside other emerging biological interventions—namely, platelet-rich plasma (PRP), gene therapy, and tissue engineering technologies.
PRP is an autologous concentrate of platelets suspended in plasma that releases growth factors such as platelet-derived growth factor (PDGF), transforming growth factor-beta (TGF-β), and vascular endothelial growth factor (VEGF). These bioactive molecules promote tissue repair and modulate inflammation at the site of injection. Systematic reviews and meta-analyses have shown that intradiscal and periarticular PRP injections can significantly reduce pain and disability scores in patients with CLBP. One meta-analysis found that PRP injections improved pain scores within 8 weeks (mean difference: –1.47; 95% CI, –2.12 to –0.81) and increased the likelihood of achieving >50% pain relief at three months compared to control groups, without a notable rise in adverse events [39].
Further, a 2023 systematic review of 40 trials reported favorable safety and efficacy outcomes in PRP-treated patients across various spinal pain sources, including discogenic and facet joint-related pain. Among 13 randomized controlled trials analyzed, 11 showed significant improvement in pain and function, supporting PRP's role as a viable alternative or adjunct to conventional therapies [40]. However, PRP’s regenerative potential is considered inferior to MSCs due to its limited cellular content and primarily short-term paracrine effects. While MSCs can replace or stimulate disc cells and contribute structurally, PRP primarily modifies the inflammatory microenvironment without addressing cell loss or disc hydration long-term [41].
Gene therapy represents another frontier in treating intervertebral disc degeneration. This strategy typically involves viral or non-viral vectors delivering therapeutic genes that modulate matrix synthesis, suppress catabolic enzymes, or reduce inflammation within disc tissues. Target genes commonly explored include SOX9, TIMP-1, and TGF-β for enhancing anabolic pathways, while RNA interference (RNAi) and CRISPR-Cas9 approaches have been applied to silence pro-inflammatory genes such as IL-1β and TNF-α [42,43].
Recent advances have focused on utilizing gene therapy to activate autophagy pathways, particularly targeting the mammalian target of rapamycin (mTOR) signaling axis. This could promote disc cell survival and matrix homeostasis in hypoxic and acidic disc environments. However, gene therapy in discogenic back pain is still largely preclinical, with issues such as precise gene regulation, transfection efficiency, and immunogenicity remaining significant translational barriers [43].
Tissue engineering strategies aim to restore disc integrity through scaffolds, cell sheets, or hydrogel-based systems. Notably, genetically modified cell sheets have emerged as promising delivery systems for regenerative gene products. These engineered constructs preserve extracellular matrix (ECM) architecture and intercellular junctions, facilitating more natural integration and signaling. They have demonstrated efficacy in regenerating cartilage, bone, and periodontal tissue, and are now being considered for spinal applications. While preclinical models show promise, safety concerns related to gene transfection methods and controlling transgene expression have delayed clinical translation [44].
Compared to MSC therapy, tissue-engineered constructs may offer better spatial control and scaffold support but lack the self-renewing and systemic paracrine versatility of live stem cells. MSCs also present a more established clinical profile with several early-phase human trials already completed in CLBP, whereas most tissue-engineering approaches are in the experimental stage.
Challenges and Limitations of MSC Therapy for CLBP
Despite promising findings in both preclinical and early clinical studies, the widespread clinical translation of mesenchymal stem cell (MSC) therapy for chronic low back pain (CLBP) faces several critical challenges. These include the lack of standardized MSC sources, inconsistencies in delivery methods and dosing, and complex regulatory and ethical landscapes governing stem cell applications.
Standardization and variability in MSC sources
One of the most pressing challenges in MSC therapy is the heterogeneity of cell populations used across different studies and clinical applications. MSCs can be derived from bone marrow, adipose tissue, umbilical cord, Wharton's jelly, and other tissues, each exhibiting unique transcriptomic, proteomic, and functional characteristics. These variations contribute to inconsistent therapeutic efficacy across trials [45]. Moreover, donor-related factors such as age, sex, health status, and comorbidities significantly influence the regenerative and immunomodulatory properties of MSCs [46]. Manufacturing inconsistencies—including differences in culture conditions, growth factor supplementation, and cryopreservation protocols—further complicate efforts to predict therapeutic outcomes [45]. The lack of universally accepted markers or potency assays for defining and validating MSC function remains a major barrier to reproducibility and regulatory approval [47].
Delivery methods and dosage optimization
Clinical outcomes from MSC therapy are also heavily influenced by route of administration, cell dose, and delivery matrices (e.g., hydrogel scaffolds, exosomes, or direct injection). Studies have employed intradiscal, perineural, and intravenous routes, each with distinct bioavailability profiles and potential for off-target effects. In many cases, insufficient homing or short-lived viability at the injury site has limited MSC efficacy [47,48]. Additionally, the optimal number of cells to achieve sustained clinical benefits is still undetermined and likely varies based on the MSC source and indication. While some trials report improvements with 10–20 million cells, others use doses up to 100 million, without a clear dose-response relationship [49]. Further complicating this issue is the lack of consensus on the number and frequency of administrations necessary for long-term therapeutic impact.
Regulatory and ethical considerations
The regulatory framework for MSC therapies is still evolving and differs significantly between countries. In the United States, MSC-based products are regulated by the FDA under the framework for human cells, tissues, and cellular and tissue-based products (HCT/Ps), requiring rigorous safety and efficacy data before approval [50]. In contrast, some countries permit conditional use or compassionate access without complete clinical validation, leading to variations in product quality and public trust. A further complication arises with the use of allogeneic MSCs, which, while offering logistical advantages, pose challenges related to immunogenicity, batch variability, and long-term safety monitoring [51].
On the ethical front, MSC therapy—particularly when derived from perinatal or embryonic tissues—faces scrutiny related to donor consent, cell origin, and religious perspectives. Although adult and autologous MSC sources reduce many of these concerns, the broader ethical landscape continues to influence funding, regulation, and public perception [52]. Ensuring transparent reporting, standardized protocols, and equitable access are essential steps toward responsible clinical translation.
Future Directions
As mesenchymal stem cell (MSC) therapy advances through early-phase clinical trials for chronic low back pain (CLBP), future research is increasingly focused on two critical areas: the development of combination therapies to enhance regenerative efficacy and long-term studies to assess durability, safety, and clinical relevance of MSC-based interventions.
Combination therapies
Emerging evidence supports the synergistic potential of combining MSCs with other regenerative modalities, particularly platelet-rich plasma (PRP) and biomaterials. In preclinical models of spinal cord injury, the co-administration of MSCs and PRP significantly enhanced axonal regeneration, increased neurofilament-positive fiber density, and reduced apoptosis compared to either therapy alone. These effects were linked to upregulation of anti-apoptotic genes (Bcl-2) and suppression of pro-apoptotic markers (caspase-3), suggesting a mechanistic synergy between MSC-secreted factors and PRP-derived growth factors [53].
Beyond PRP, studies have explored biomaterial scaffolds and hydrogel matrices as delivery platforms to improve MSC retention, survival, and localization at the injury site. Such scaffolds not only serve as structural support but also modulate the local microenvironment, potentially enhancing MSC-mediated immunomodulation and matrix synthesis [54]. The convergence of MSC therapy with neuromodulation, physical rehabilitation, and gene editing technologies represents an additional frontier. These multimodal approaches may amplify therapeutic effects, facilitate neuroplasticity, and optimize recovery pathways in degenerative disc disease and related neuropathic conditions [55].
Long-Term safety and efficacy studies
While short-term outcomes from early-phase clinical trials are promising, longitudinal data remain scarce. Most published studies report outcomes up to 12–24 months, with few addressing whether observed structural improvements (e.g., disc hydration or annular repair) translate to sustained functional recovery or slowed disease progression. Furthermore, the durability of MSC effects—especially with respect to repeated dosing or in aging or comorbid populations—remains uncertain [56].
Ensuring long-term safety is equally critical. Though MSCs have demonstrated favorable short-term safety profiles in clinical trials, theoretical risks such as ectopic tissue formation, immune sensitization, or genomic instability require ongoing surveillance. To address this, new safety evaluation systems have been developed based on current regulatory frameworks, integrating quality control metrics such as identity, potency, viability, and sterility testing. A study on human umbilical cord MSCs (HUCMSCs) noted no severe adverse reactions over a one-year follow-up in 225 patients, supporting their continued investigation while also emphasizing the need for broader population-level validation [56].
Additionally, large-scale GMP-compliant manufacturing must balance scalability with quality control. Advances in bioreactor systems, hypoxic culture conditions, and serum-free media are being explored to maintain MSC phenotype and therapeutic function during expansion [57]. These industrial strategies are pivotal for producing consistent, high-quality cell products suitable for late-phase trials and regulatory approval.
Summary & Conclusion
Key findings
Mesenchymal stem cell (MSC) therapy has emerged as a promising regenerative strategy for addressing the multifactorial pathology of chronic low back pain (CLBP), particularly in cases involving intervertebral disc degeneration. Preclinical studies consistently demonstrate that MSCs promote disc matrix restoration, suppress inflammation, and reduce neuropathic pain via paracrine signaling mechanisms and immunomodulation [23,24,29]. These effects are mediated by the secretion of bioactive molecules, including growth factors and extracellular vesicles, which enhance cell survival, matrix synthesis, and neuroprotection within the degenerative spinal microenvironment [25,26].
Early-phase human clinical trials report favorable safety profiles and improvements in patient-reported outcomes, including pain scores, functional disability indices, and imaging markers such as disc height and hydration. Autologous and allogeneic MSCs, derived from sources such as adipose tissue and bone marrow, have both demonstrated efficacy, with some studies reporting clinically significant pain reduction and decreased reliance on analgesics over 12-month follow-up periods [34-36]. While results vary based on cell source, dose, and delivery method, the majority of trials underscore MSCs’ potential to address the underlying pathophysiology of CLBP rather than solely alleviating symptoms.
Combination therapies—such as co-administration with platelet-rich plasma (PRP) or biomaterials—have shown synergistic effects in preclinical models, enhancing MSC survival, retention, and regenerative impact [53,54]. Furthermore, long-term studies suggest sustained clinical benefit without serious adverse events, though data remain limited beyond two years.
Current gaps in research
Despite growing evidence supporting the therapeutic potential of mesenchymal stem cells (MSCs) in chronic low back pain (CLBP), several critical gaps in the current body of research hinder widespread clinical translation. One of the foremost limitations is the lack of standardized protocols across studies. Variability in MSC sourcing, isolation, expansion, and characterization leads to heterogeneity in cellular potency, viability, and secretory profiles, making it difficult to compare results or establish universally accepted therapeutic benchmarks [45,47].
Additionally, optimal delivery routes and dosing regimens remain poorly defined. While intradiscal, intravenous, and scaffold-based delivery methods have all shown promise, few studies directly compare their efficacy, and even fewer evaluate the kinetics of MSC retention, survival, or biodistribution in human subjects [48,49]. The dose–response relationship is similarly unclear, with trials reporting therapeutic benefits across a wide range of cell concentrations but without consensus on ideal thresholds for efficacy or safety.
Furthermore, while short-term outcomes are encouraging, there is a notable absence of long-term data assessing durability of pain relief, functional restoration, or structural disc regeneration beyond 24 months. The potential for delayed adverse effects, such as ectopic tissue formation or immune sensitization—particularly with allogeneic cell products—remains insufficiently explored [46,56].
Finally, few studies incorporate large-scale, multicenter trial designs or comparative analyses against existing standard-of-care treatments. As a result, the relative cost-effectiveness and clinical utility of MSC therapy in broader patient populations are still undetermined. Addressing these gaps through rigorous, standardized, and longitudinally designed clinical trials will be essential to transition MSC-based interventions from investigational procedures to mainstream therapeutic options for CLBP.
Conclusion
Chronic low back pain (CLBP) remains a leading cause of disability worldwide, with current treatments often limited to symptomatic relief rather than true biological repair. As the field of regenerative medicine continues to evolve, mesenchymal stem cells (MSCs) offer a compelling paradigm shift—targeting the underlying disc degeneration, inflammation, and neuropathic components that drive chronic pain. Beyond their capacity to differentiate, MSCs exert broad immunomodulatory and trophic effects that align well with the multifactorial pathology of CLBP.
With accumulating evidence from preclinical and early-phase clinical studies, MSCs are increasingly recognized not just as a therapeutic option, but as a potential cornerstone of future biologic strategies for spine care. Their use in combination therapies—such as with platelet-rich plasma (PRP), biomaterials, or gene modulation—represents an exciting and rational extension of current approaches, potentially amplifying therapeutic impact through synergy. Such integrated strategies may not only improve cellular retention and survival but also enhance regenerative and neuromodulatory responses within the hostile environment of the degenerative disc.
The field is now approaching a critical juncture where the integration of regenerative therapy into standardized clinical algorithms for CLBP must be guided by robust, long-term evidence and carefully designed translational models. As research continues to bridge laboratory innovation with bedside application, MSC-based therapies hold promise to transform the management of CLBP—from palliative symptom control to true biological restoration. With continued interdisciplinary collaboration, technological refinement, and patient-centered investigation, regenerative approaches may soon redefine the standard of care for millions affected by spinal degenerative disease.