The Global Health Crisis of Sickle Cell Disease and the Impact of Stem Cell Therapy as a Viable Treatment
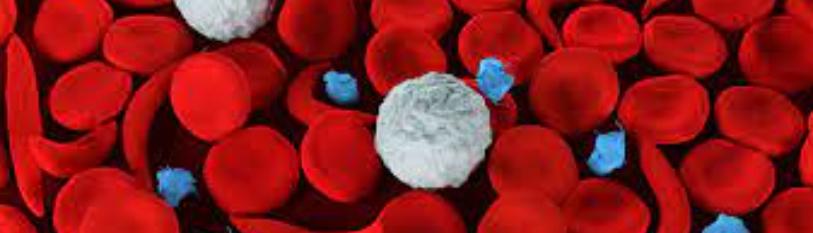
Hannah Chappell and Vincent S. Gallicchio*
Department of Biological Sciences, College of Science, Clemson University, Clemson, SC 29636
*Corresponding author: Vincent S. Gallicchio, Department of Biological Sciences, College of Science, Clemson University, Clemson, SC 29636
Citation: Chappell H , Gallicchio VS. (2022) The Global Health Crisis of Sickle Cell Disease and the Impact of Stem Cell Therapy as a Viable Treatment. J Stem Cell Res. 3(1):1-13.
Received: January 26, 2022 | Published: February 14, 2022
Copyright© 2022 genesis pub by Chappel H, et al. CC BY-NC-ND 4.0 DEED. This is an open-access article distributed under the terms of the Creative Commons Attribution-Non Commercial-No Derivatives 4.0 International License., This allows others distribute, remix, tweak, and build upon the work, even commercially, as long as they credit the authors for the original creation.
DOI: https://doi.org/10.52793/JSCR.2022.3(1)-27
Abstract
Sickle cell disease is an inherited hematological disorder where the presence of this gene can impact the function of hemoglobin, causing it to polymerize and form the abnormal sickle red blood cells. Vascular occlusion is usually associated with this disease, as the abnormal shaped red blood cells are unable to move throughout microvasculature of the body and get trapped in vessels, causing a pain episode for patients which is referred to as being one of the most prominent and agonizing symptoms of the disease. Throughout the investigation of various forms of treatment for this disease, specific methods stand out from others with successful implementation or promising ongoing results. Hydroxyurea stands out among these various forms as a frontrunner as it has been investigated in low-resource countries such as Uganda and highlights several factors that make it low-cost and easily distributable. Stem cell therapy and CRISPR-gene editing technology are also promising forms of treatment, although there are questionable success rates and numerous obstacles preventing them from being highly distributed. By implementing these various forms of treatment in sub-Saharan Africa, where the prevalence of sickle cell disease is at its highest, we can alleviate the global health disparity of this disease in areas with less resources and inadequate health care attention. The biggest obstacle the world faces in regard to sickle cell disease is how best to optimize these methods of treatment by making them accessible and effective in the areas that need them the most.
Keywords
Sickle cell disease; Stem cell; Hydroxyurea; CRISPR-tech; Global health
Introduction
What is Anemia?
Anemia is a condition which impacts the amount of healthy red blood cells that carry oxygen throughout the body to maintain ample homeostatic conditions. The true disease of anemia is low hemoglobin, which is when your body doesn’t make enough red blood cells or destroys them. Typical symptoms of mild anemia cause one to feel tired and weak, with other symptoms ranging from paleness of the skin, shortness of breath/chest pain, and cold extremities. Anemia can be temporary or long-term, with a variety of treatment including supplements, variations in diets, and medical procedures [1].
Anemia can take two general forms, congenital or acquired [2]. Congenital anemia is present at birth and has a variation of types which range from mild to severe throughout one’s lifetime. Acquired anemia is a condition which develops later in life and occurs in individuals that once had a normal red blood cell system [3].
Sickle Cell Anemia
This form of anemia can become very serious and sometimes fatal [1]. Sickle Cell Anemia occurs from a defective form of hemoglobin which results in abnormal red blood cell shape that dies prematurely, resulting in a shortage of red blood cells [1]. The sickle cell blood shape resembles a crescent moon shape, which becomes stiff and sticky and blocks blood flow through the vessels [4]. These abnormal red blood cells cause pile ups in the blood vessels, preventing organs from getting the oxygen they need and resulting in an amplitude of issues [4]. Symptoms of sickle cell disease include episodes of pain from the block ups in blood vessels surrounding the chest, abdomen, bones, and joints, swelling of extremities due to the lack of blood flow to these areas, frequent infection from damaged organs, delayed growth, or puberty due to the lack of oxygen impeding on normal growth development, and vision problems resulting from the blood flow blockage to the optic nerve [5].
The genetics of this disease is interesting in that the homozygous and heterozygous individuals result in different phenotypic expressions. Both parents need to carry the gene in order for the disease to be inherited. Homozygous individuals develop sickle cell anemia, while heterozygous individuals are proven beneficial against fighting malaria [6]. High prevalence of sickle cell anemia is seen with the heterozygous genetic makeup in areas where levels of malaria are high [6]. High incidence areas are due to the lack of awareness among both local and public health policy makers [7]. Sickle cell disease targets certain ethnic groups including Hispanic americans from central and south America, people of middle eastern, Asian, Indian, and Mediterranean descent, and most seen in people of African descent and the sub-Saharan Africa region [4].
What is Global Health?
Global health is generally defined as the health issues that surpass national boundaries and require global interference and cooperation [8]. While public health focuses on health issues within a specific country or community, global health is action oriented and puts emphasis upon the need of intervention on a global scale [8]. The highest level of global health disparity is seen throughout differences in geography across the globe. Usually, low-income countries face the largest global health differences when compared to high income countries because wealthier countries can spend more money on healthcare [8,9]. The allocation of healthcare resources varies vastly among geographical regions, where some areas are highly impacted by diseases that are unknown to other areas of the world [10]. To demonstrate the severity of the global health disparity, I will be investigating sickle cell disease and how its impact is spread globally. The major global component of sickle cell disease is seen through the allocation and distribution of treatment resources and how new technology is providing vast breakthroughs in treatment of this disease, while only being provided in certain areas of the world that can afford it rather than being based on need.
Discussion
Origin and pathway of Sickle Cell Anemia
The first case of sickle cell anemia in western medicine was determined by James Herrick in 1910 through his discovery of abnormal hemoglobin cells in a 20-year-old Indian student [11] (Figure 1).
Figure 1: Advances in Sickle Cell Disease: a timeline tracking the progress of treating sickle cell disease since its discovery in 1910 [12].
This figure effectively outlines the advances that have been made since this discovery and the ways in which the methods of treatment for this disease are growing and evolving, especially over the last decade. Upon Herrick’s groundbreaking discovery, Pauling, Itano, Singer, and Wells defined the cause of the odd shaped hemoglobin to be the result of a point mutation in the gene that codes the β chain of hemoglobin [13,14]. This mutation caused a single amino acid substitution of valine for glutamine, and when found on both chromosomes in a patient, would result in sickle cell hemoglobin, abbreviated HbS [13].
The normal human hemoglobin consists of two α chains and two β chains, resulting in four total globin chains and abbreviated as HbA [13]. Abnormal human hemoglobin is seen impacting the globin chains, where most abnormalities are seen in the β chain rather than the α chain [13]. The point mutation responsible for causing sickle cell hemoglobin occurs at the sixth position of the β chain [13]. This point mutation causes the replacement of glutamine with valine, which has a high affinity to bond to other hemoglobin in another chain when deoxygenated [13]. When this bonding occurs, the result is a twisted, ropelike structure with two complete hemoglobin chains, with bonding between the β chains [13]. This process occurring when the mutated hemoglobin binds to other hemoglobins in its deoxygenated state is known as polymerization, and causes distortion of the RBC molecule, creating sickle shaped cells, as well as increased rigidity of the molecule [13]. Increased rigidity of a RBC molecule hinders its ability to travel through blood vessels of the body, introducing the possibility of vascular occlusion to occur [15].
The process of polymerization is responsible for catalyzing the movement of certain ions across the membrane including sodium, potassium, magnesium, and calcium [13]. The movement of these ions across the membrane causes the transport of water to occur, overall dehydrating the RBC molecule [13]. The dehydration of RBC molecules signals for a higher concentration of hemoglobin molecules in the cell [13]. The dehydration of RBC molecules is also followed by an increased viscosity of the blood which slows the rate of blood flow due to the thickness of its contents struggling to move through small capillaries [13]. An increased concentration of hemoglobin molecules will accelerate the rate of polymerization, which illustrates how the sickle shape RBC molecules are continuously produced and can begin to heavily impact the body’s normal functioning [13]. The polymerization of this abnormal HbS molecule is the primary player in the pathogenesis of the sickle cell disease.
Vascular Occlusion because of sickle cell anemia
One result of the polymerization of mutated hemoglobin molecules is vascular occlusion, where blood is no longer able to pass through a blood vessel [13]. Prior to polymerization, RBCs carrying HbS are very flexible, but gain rigidity when they deoxygenate and polymerize, virtually trapping these cells in the vessels of blood circulation [15]. Vaso-occlusion of the RBCs depends upon both the delay time that occurs between deoxygenation and polymerization of HbS and the rate of blood flow through a given blood vessel [13]. Rather than being an instantaneous process, the polymerization of HbS maximizes after a delayed amount of time, allowing for RBCs to escape into larger blood vessels before vasoconstriction occurs while entering a capillary or other form of microvasculature in the body [15]. Rate of blood flow is also an important factor in the ability of sickle hemoglobin to polymerize and entrap RBCs because when blood flow decreases, the transit time of RBCs escaping into larger blood vessels is prolonged, thus allowing for polymerization to occur before the RBCs can make it out of microvasculature [15].
Hydroxyurea Treatment
Sickle cell disease requires lifelong treatment and, while there is no end all cure for sickle cell disease, there have been methods of maintenance discovered as this disease has been better understood since its first occurrence. Hydroxyurea is a powerful medication that can be taken once a day to maintain episodes of pain and limit the frequency that these episodes occur [16]. Hydroxyurea is most used in SCA patients due to its ability to reduce the number of neutrophils and reticulocytes in marrow as well as the number of platelets, which are indicators of inflammation [16]. An elevated WBC count is found to be closely correlated with mortality in SCA patients, so lowering this number is highly beneficial in the outcomes of this disease [16]. Neutrophils and reticulocytes promote vascular occlusion, so reducing these numbers reduces the probability of blockage in major vessels, which corresponds with the pain crisis experienced by infected patients [16].
A study conducted by DeBaun illustrates how hydroxyurea was proven superior in treating children with SCA that were prone to strokes during the depleted blood supply of the COVID-19 pandemic [17]. Starting low-dose hydroxyurea treatment for children already receiving blood transfusions for primary or secondary stroke prevention was defined as advantageous through this study because hydroxyurea has a low risk for myelosuppression, where bone marrow activity is decreased [18]. This enables the patient to receive this form of treatment without having to undergo continuous laboratory surveillance beyond the testing for pregnancy [17]. Another advantage of administering low-dose hydroxyurea to these patients is that there is little to no lag time of clinical benefit when the patient halts blood therapy and begins hydroxyurea treatment [17]. Thirdly, hydroxyurea treatment decreases the incidence rate of vaso-occlusive pain episodes of children and adults with SCA in low-resource areas that lack laboratory surveillance [17].
Russell Ware further highlights how hydroxyurea therapy is proven beneficial in children and adolescents to prevent vaso-occlusive pain crises as well as illustrating the extensive timeline of hydroxyurea and how its use has been effectively maximized over the past several decades [19]. Within this figure is the BABY HUG trial, a trial that highlighted the benefits that hydroxyurea had on acute pain crises in infants and young children [20]. Long-term clinical benefits have also been observed using this drug with less hospitalizations and increased quality of life overall [19]. Children as young as 2 years old and adolescents are safely started at 20 mg/k/d administered orally with blood counts every 4 weeks [19]. If myelosuppression is not recognized in these patients, their doses can be increased by 5 mg/k/d to receive maximal laboratory benefits within 6 to 8 months on the treatment [19] (Figure 2). A study conducted by Chandy, et al. in June of 2020 focused on the dose escalation of hydroxyurea and its impacts on patients in sub-Saharan Africa compared to that of fixed doses of hydroxyurea [21]. 94 children between ages 4-6 received a fixed dose of hydroxyurea at 20 mg/k/d and 93 children between ages 4-6 received an elevated dose of hydroxyurea at 30 mg/k/d, as illustrated in the figure below [21].
Figure 2: Clinical Efficacy of Hydroxyurea: delineates the various studies and trials that established hydroxyurea as such a powerful method of treatment for sickle cell disease [20].
The results displayed that 86% of the dose escalation group reached fetal hemoglobin levels of 20% or more after 24 months, while only 37% of the children in the fixed-dose group achieved this outcome [21]. This study not only highlights the positive impacts of hydroxyurea in general, but specifically the ability for increasing dosage to prove clinical efficacy in a shorter period.
A current clinical trial of note is underway to evaluate the effectiveness of hydroxyurea in sickle cell vasculopathy of newborns, a potential brain injury caused by the blood vessel damage corresponding with sickle cell anemia [22]. SCV is associated with a high risk of stroke, and this study intends to assess the ability of hydroxyurea administration to prevent, stabilize, or improve the risks of SCV [22]. This trial is sponsored by Global Health Uganda LTD and consists of 270 participants ranging from ages 3-9 residing in Uganda, standing out as being the first Africa-based trial to alleviate the global health impacts of anemia [22]. The ability for hydroxyurea to produce quick results in the form of a low-cost oral medication places it among the most ideal methods of treatment for sickle cell disease. Hydroxyurea has been analyzed and vastly understood for over 25 years to become one of the leading methods of treatment in the race to maintain and overcome the crisis of sickle cell anemia (Figure 3).
Figure 3: Hydroxyurea Dose Escalation: results of changing hydroxyurea dosage in Sub-Saharan Africa [21].
Stem Cell Therapy Treatment
Stem cell therapy is among the few forms of treatment for sickle cell anemia that has curative intent. The idea is that fostering bone marrow from a healthy patient, usually a sibling that has matching bone marrow, and transplanting it into the patient infected with sickle cell hemoglobin will allow for healthy hemoglobin to be produced [23]. Before the transplant, bone marrow cells are destroyed in the infected patient to have the immune defenses weakened to avoid the rejection of the donor marrow, which gets injected through the veins of the recipient patient [23]. The donor bone marrow replaces the entirety of the infected patient’s marrow, allowing for the immune system to be restored and normal red blood cells to be produced [23]. It has been found that sibling matched stem cell transplantation has 90% overall survival and 80% event-free survival [24]. However, only 25-30% of patients have a matched sibling donor, making it vital for stem cell therapy to be investigated through alternative routes [24].
Through studies performed by Lucarelli, et al. stem cell transplant has provided disease-free survival of about 85% in patients that receive the transplant [25]. Cord blood transplantation is a viable option for those that can’t find suitable donors, but there have been recorded higher occurrences of GVHD through this form of transplantation [25]. Through evaluation of the Rome experience, where 11 SCA patients ranging from 6-12 years old were divided amongst groups based on the different drugs they were administered pre-transplant, it was evident that donor engraftment in the recipient patients was highly prevalent with various forms being seen in all 11 patients [25]. However, 10 out of the 11 patients survived without sickle cell disease with full donor chimerism in lymphoid and myeloid lineages [25].
The current obstacle researchers are trying to overcome with stem cell therapy is battling the lack of HLA-matched siblings as donors which, in the US, is available to less than 15% of patients with sickle cell disease [12]. A study performed by Fitzhugh, et. al. introduced matched unrelated donors (MUD) through the discovery that only 20% donor myeloid chimerism, or engraftment from donor cells to recipient cells, is needed to reverse the sickle phenotype [20]. This idea is based on the relationship between RBC lifespans of the donors and recipients and showed high sensitivity to this correlation [20]. While displaying promising results, this research team has not found a perfect combination of RBC life spans between matched unrelated donors to cure sickle cell disease in the infected patient, although this research is ongoing [20].
Stem cell therapy is one of the only methods of treatment of sickle cell disease that can provide a cure, however, there is a current struggle with finding infected patients the perfect match for stem cell transplantation, especially for African and African American populations, in a form of transplantation that can definitively alleviate the patient of their sickle cell disease for the remainder of their lifetime [12].
Gene therapy
Among the newest methods of sickle cell treatment is CRISPR gene editing which uniquely uses the infected patient’s own stem cells to replace the mutated genes [26]. This powerful method of treatment is found so effective because you are using the infected patient’s own cells, so there is no chance of graft versus host disease (GVHD), which has proven to be a heavy possible complication in stem cell treatment [26]. CRISPR technology takes an innovative and different approach to battling sickle cell disease by increasing levels of fetal hemoglobin instead of directly targeting the disease-causing mutations [27]. Fetal hemoglobin is only made in the womb and can replace defective hemoglobin in adults [27]. The first step of CRISPR gene editing treatment is to harvest stem cells from the infected patient and edit the genomes [27]. Chemotherapy eliminates the defective blood cells from the patient’s body, and then billions of gene edited blood stem cells are put back into the bloodstream via IV [27]. The intended result is for the gene edited blood stem cells to create a new stem cell population in the bone marrow which will produce fetal hemoglobin that replaces the defective adult hemoglobin [28]. This form of CRISPR-gene editing is currently undergoing a clinical trial with a patient named Victoria Gray from Nashville, Tennessee who began her participation in July of 2019 [27]. There has been evidence that the gene-edited blood cells successfully created a new population in her bone marrow and produced fetal hemoglobin, and the only immediate side effects recognized were from the chemotherapy performed [27].
A unique clinical trial is underway through the University of California Consortium made up of researchers from University of California San Francisco, University of California at Berkeley, and University of California Los Angeles [26]. This trial will last four years and involves nine patients, six adults and three adolescents with severe sickle cell disease residing in Los Angeles and Oakland, California [26]. This research group is taking an innovative approach to gene editing by directly targeting the mutated beta-globin gene in the diseased patient’s own cells to repair the sickle cell disease at the root of the cause [28]. The group has developed CRISPR_SCD001, which is a patient specific blood stem cell therapy that will stimulate the repair of the sickle mutation [28]. This trial will involve the patient’s blood stem cells to be extracted and sent to the UCLA lab for electroporation, where the cells are exposed to electrical impulses that cause them to develop pores in their membranes [28]. These pores allow for the CRISPR gene editing tools to be inserted into the cell and target the cell’s nuclei, where they reverse the sickle cell mutation before the blood stem cells are reintroduced to the patient [28]. By correcting this mutation in at least 20% of the patient’s blood stem cells, the goal is to outcompete the native stem cells and produce corrected red blood cells throughout circulation [28].
Another recent trial that has proven successful is led by Dr. Donald Kohn and Dr. Gary Schiller, funded by the California Institute for Regenerative Medicine, and involves a new gene being introduced into the blood stem cells to correct the sickle cell mutation [28]. This method of treatment involves the removal of the blood stem cells from the infected patient and the addition of a modified version of the beta-globin gene that is mutated in sickle cell patients [29]. This modified beta-globin gene targets the cell’s DNA and blocks sickling from occurring [30]. To get the modified beta-globin gene into the cell’s DNA, Kohn utilizes a viral vector, which is a virus that can introduce information to a cell’s nucleus without causing infection [30]. Upon testing of several different vectors, “Mini G” was the most effective vector due to its small size and ability to quickly enter the cell’s nuclei as well as being more cost efficient [30]. Evie Junior is a 27-year-old sickle cell patient participating in this trial and showed 70% of his blood cells containing the new corrected gene three months after treatment [29]. Junior also hasn’t had any pain crises since undergoing the treatment and noticed an overall better quality of day-to-day life [30].
The innovative and high success rate of CRISPR-gene editing tools is revolutionizing all levels of genetic medicine and indicates promising outcomes for curing sickle cell disease. However, to target sickle cell disease in the areas where it is most prevalent, there is a battle of making this method of treatment affordable and able to be widely distributed in low-resource countries.
Global health trend of sickle cell anemia
While the treatment methods of sickle cell anemia are ongoing and growing in effectiveness, there is also a continuous struggle of making these methods of treatment available to the areas of the world that need them the most. A common theme throughout the research of sickle cell anemia involves its impact and prevalence throughout sub-Saharan Africa. While we see the presence of sickle-cell disease in the U.S., it is far more prevalent and detrimental in the sub-Saharan African region of the world, and it is vital that researchers tailor therapeutic options that can be accessed by the areas that suffer from this disease the most [12].
Research done by Batina, et al. effectively highlights the weight of the disparity among access to health care across the world [31]. The World Health Organization (WHO) estimated that 70% of deaths due to sickle cell disease in sub-Saharan Africa are preventable by access to early diagnosis, information, and education [31]. This research team conducted a study in 2010 of 158 sickle cell patients that were consulted about their disease in one of the general hospitals in the city of Kisangani in the Tshopo province of the Democratic Republic of Congo [31]. Of the 158 patients studied, 44.9% believed that their disease was an outcome of devil origin or divine curse [31]. 93.6% of the patients found they were unable to afford health care associated with sickle cell disease [31]. This study provides vital insight into the methods of intervention that will be most successful in tackling this disease throughout low-resource areas.
Hsu, et al. conducted a study that identified that of the 401,000 babies that are born with sickle cell disease globally per year, 70-75% of them are in sub-Saharan Africa [32]. This study focused on the value of sustained neonatal screening for sickle cell disease and ways in which this is achievable [32]. Of high importance in this research was the misallocation of resources and global funding to areas that were “country priorities,” with healthcare officials lacking an understanding of the correlation between sickle cell disease and various infectious and non-communicable diseases that were crippling the region [32]. This research highlights that, while countries may still struggle with providing health care associated with sickle cell disease, the awareness of the diagnosis can cause the family to react differently when their infected child suffers from fevers or episodes of pain [32].
The first step in combating the global health disparities for sickle cell disease begins with education within sub-Saharan Africa of not only the patients, but the patient’s families as well as the community as a whole. Another defining aspect of the inconsistency of managing this disease where it affects individuals the most is associated with the differing cultural views that are upheld, specifically the traditional healing methods that they trust in [31]. Incorporating traditional healers into modern medical approaches is vital in further educating these populations on sickle cell disease. Several small changes such as neonatal screenings can impact the overall spread and severity of sickle cell anemia. While there is an assortment of new methods of treatment being cultivated throughout the world, they are impractical if they cannot be distributed and sustained in the areas that suffer from the highest numbers of the disease and the most severe forms of the disease.
Summary
Sickle cell anemia is an overwhelming disease that has interesting evolution and genetic aspects. Through its defection of the hemoglobin molecule, this disease can reach very severe stages and create a poor quality of life for patients of all ages. Once sickled hemoglobin polymerizes, its effects are widespread throughout the circulation of the body through the abnormal shape of the red blood cells, impacting the rate and ability for blood to flow through various levels of vasculature. Blockage within major vessels is followed by episodes of pain crises which are sudden and traumatic for the patients and maintaining treatment of this symptom as well as a variety of other pervasive symptoms has captivated researchers across the world for decades.
The various methods of treatment surrounding this disease take on vastly different forms as evaluated through several past and current clinical studies. Hydroxyurea stands out as a frontrunner for its ability to be distributed at low costs and high availability as well as showing promising results when doses are enhanced in patients. A unique curative treatment of sickle cell disease is stem cell therapy, although there are shockingly low numbers of donors being matched to recipients in need of transplantation. And finally, gene editing is a captivating modern form of medical technology that takes genetic research to the next level. By targeting the mutated gene in sickle cell disease to reverse its effects is non-invasive for the recipient and prevents any issues of rejection. Among other uncertainties surrounding this new method of treatment is the ability for it to be distributed to low-income countries experiencing the high prevalence of sickle cell disease.
The major results found in the studies surrounding the methods of treatment of hydroxyurea, stem cell therapy, and CRISPR-gene editing technology highlight the ways in which their effects can be optimized and implemented in the high-prevalence sickle cell disease regions of the world. Hydroxyurea shows the most promising and credulous results, with its low-cost and success in countries such as Uganda. Stem cell therapy, while a powerful curative option, faces the obstacle of trying to find bone marrow donor matches and other forms of this therapy that don’t rely on perfect matches are in the early stages of development. CRISPR-gene editing allows for the possibility to correct the mutation that is responsible for sickle cell disease in an individual, however this is the most high-cost option and is also ongoing in various clinical studies to prove its clinical efficacy.
Conclusion
In conclusion, there is hope in the fight to not only cure sickle cell disease but provide maintenance and relief of the disease’s most painful symptoms that impact a patient’s day-to-day life. The most concrete evidence surrounding the effective forms of treatment of sickle cell disease highlights hydroxyurea to be the most reliable and accessible drug that reduces major symptoms of this disease and overall maintains it while not curing it. Michael DeBaun conducted a study on how hydroxyurea could be used in place of blood transfusions for children that were prone to strokes due to sickle cell disease. Russel Ware’s study highlights how hydroxyurea targets vaso-occlusive events in children due to its lack of myelosuppression. A study conducted by Chandy, et al. illustrates how hydroxyurea shows success in sub-Saharan Africa and its dosage can be enhanced to further advance its effects in a shorter amount of time.
Another effective form of treatment that was investigated includes stem cell therapy, which has curative intent but faces a struggle of finding perfect bone marrow matches for patients with sickle cell. Stem cell therapy through sibling donor transplantation shows an overall survival rate of 90%, however only 25-30% of sickle cell patients are eligible for sibling donor transplants, requiring other forms of this therapy to be implemented. Lucarelli, et al. conducted a study on 11 individuals to implement an HLA-matched stem cell transplantation where they found 10 of the 11 individuals survived without sickle cell disease but all patients experienced GVHD in some form [25]. While stem cell therapy has a high chance of curing patients, there are several different factors that can cause postoperative complications, and this form of treatment is still ongoing clinical studies in order to be strongly refined for the future.
CRISPR-gene editing is the third method of treatment that has been found to be promising and effective in its ability to correct the mutation which causes sickle cell disease to occur. This is the newest method of treatment with ongoing clinical studies that have little results currently, however, the overall goal is to correct the gene in 20% of the patient’s cell population to outcompete the native cells and correct sickle cell disease from the root of the source [28]. Victoria Gray is a patient of a current clinical study which intended to replace the defective hemoglobin with fetal hemoglobin, and her post-treatment analysis shows that a new cell population has been created with high levels of fetal hemoglobin. The study conducted by Kohn and Schiller utilizes a viral vector to target the DNA in the nucleus of sickle cells and prevent sickling from occurring. Evie Junior is a participant in this trial and is showing promising results with 70% of his blood cells containing the corrected gene. This method of treatment can revolutionize the healing of sickle cell disease; however, it requires high-cost technology and is still in very early stages of development.
While revolutionary efforts are being made in the treatment of this disease, there is a relentless struggle of understanding the ways in which this disease can be alleviated of its global health disparities. Global health studies conducted specifically throughout sub-Saharan Africa continue to highlight the unfortunate inconsistency that exists between the transformative breakthroughs in methods of treatment and the inability to implement these methods in a sustainable and successful fashion in the areas where they are needed the most.
References
- https://www.mayoclinic.org/diseases-conditions/anemia/symptoms-causes/syc-20351360?sa=U&ved=0CBoQFjAB
- https://medlineplus.gov/genetics/condition/congenital-dyserythropoietic-anemia/
- https://rarediseases.org/rare-diseases/anemia-hemolytic-acquired-autoimmune/
- Global Blood Therapeutics Inc. (n.d.) (2021)What is sickle cell. Sickle Cell Speaks.
- https://www.mayoclinic.org/diseases-conditions/sickle-cell-anemia/symptoms-causes/syc-20355876
- https://www.sciencedaily.com/releases/2011/04/110428123931.htm
- https://onlinelibrary.wiley.com/doi/abs/10.1111/ijlh.13023
- https://doi.org/10.3402/gha.v3i0.5142
- https://www.pgpf.org/blog/2020/07/how-does-the-us-healthcare-system-compare-to-other-countries
- Rice N, Smith PC. (2001) Ethics and Geographical Equity in health care. Journal of Medical Ethics, 27(4):256-61.
- Savitt TL, Goldberg MF. (1989) Herrick's 1910 case report of Sickle Cell Anemia: the rest of the story. Jama. 261(2):266-71.
- Salinas Cisneros G, Thein SL. (2020) Recent advances in the treatment of sickle cell disease. Frontiers in Physiology. 11:435.
- Lonergan GJ, Cline DB, Abbondanzo SL. (2001) Sickle cell anemia. RadioGraphics. 21(4):971-94.
- PoMBURG TA, HA S. SJ & WELLS, IL. (1949). Science.;110:543.
- Veluswamy S, Shah P, Denton C, Chalacheva P, Khoo M, & et al. (2019). Vaso-occlusion in sickle cell disease: Is autonomic dysregulation of the microvasculature the trigger? Journal of Clinical Medicine. 8(10):1690.
- Agrawal RK, Patel RK, shah V, Nainiwal L, Trivedi B. (2013). Hydroxyurea in sickle cell disease: Drug review. Ind J Hematol Blood Transfus. 30(2):91-6.
- DeBaun MR. (2020) Initiating adjunct low-dose hydroxyurea therapy for stroke prevention in children with SCA during the covid-19 pandemic. Blood. 135(22):1997-9.
- https://www.cancer.gov/publications/dictionaries/cancer-terms/def/severe-myelosuppression.
- Ware RE. (2010) How I use hydroxyurea to treat young patients with sickle cell anemia. Blood. The Journal of the American Society of Hematology. 115(26):5300-11.
- Fitzhugh CD, Cordes S, Taylor T, Coles W, Roskom K, and et al. (2017). At least 20% donor myeloid chimerism is necessary to reverse the sickle phenotype after Allogeneic HSCT. Blood. The Journal of the American Society of Hematology. 130(17):1946-8.
- John CC, Opoka RO, Latham TS, Hume HA, Nabaggala C, and et al. (2020). Hydroxyurea dose escalation for sickle cell anemia in Sub-Saharan Africa. New England Journal of Medicine. 382(26):2524-33.
- https://www.clinicaltrials.gov/ct2/show/NCT04750707.
- https://www.uofmhealth.org/health-library/hw254074.
- Tanhehco YC, Bhatia M. (2019) Hematopoietic stem cell transplantation and cellular therapy in sickle cell disease: where are ew now? Current Opinion in Hematol. 26(6):448-52.
- Lucarelli G, Isgro A, Sodani P, Gaziev, J. (2012) Hematopoietic stem cell transplantation in thalassemia and sickle cell anemia. Cold Spring Harbor Perspectives in Medicine. 2(5):a011825.
- https://www.verywellhealth.com/crispr-technology-fda-sickle-cell-disease-5179133.
- https://innovativegenomics.org/news/crispr-clinical-trials-2021/.
- https://www.ucsf.edu/news/2021/03/420137/uc-consortium-launches-first-clinical-trial-using-crispr-correct-gene-defect?utm_source=ucsf_tw&utm_medium=tw&utm_campaign=2021_CRISPRSickleCellStudy.
- https://stemcell.ucla.edu/Evie.
- https://stemcell.ucla.edu/news/ucla-researcher-receives-nih-grant-develop-more-affordable-gene-therapy-sickle-cell-disease.
- Batina SA, Kambale PK, Sabiti MP, Kayembe CT, Gulbis B. (2017) Barriers to healthcare for sickle cell disease patients in the Democratic Republic of Congo. African J Health Issues. 1(1):3-9.
- Hsu L, Nnodu OE, Brown BJ, Tluway F, King S,and et al. (2018) White Paper: Pathways to progress in newborn screening for sickle cell disease in Sub-Saharan africa. Int J Trop Dis Health. 6(2).