The Efficacy of Autologous Stem Cell Transplants to Treat High Risk Neuroblastoma
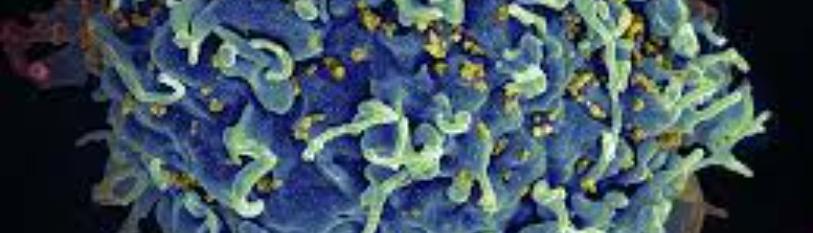
Caitlyn Murphy and Vincent S. Gallicchio*
Department of Biological Sciences, College of Science, Clemson University, Clemson, SC 29636
*Corresponding author: Vincent S. Gallicchio, Department of Biological Sciences, College of Science, Clemson University, Clemson, SC 29636
Citation: Murphy C, Gallicchio VS. (2022) The Efficacy of Autologous Stem Cell Transplants to Treat High Risk Neuroblastoma. J Stem Cell Res. 3(1):1-17.
Received: January 15, 2022 | Published: February 03, 2022
Copyright© 2022 genesis pub by Murphy C, et al. CC BY-NC-ND 4.0 DEED. This is an open-access article distributed under the terms of the Creative Commons Attribution-Non Commercial-No Derivatives 4.0 International License., This allows others distribute, remix, tweak, and build upon the work, even commercially, as long as they credit the authors for the original creation.
DOI: https://doi.org/10.52793/JSCR.2022.3(1)-26
Abstract
High Risk Neuroblastoma is a pediatric cancer that arises from immature nerve cells. While low and intermediate risk neuroblastomas remain curable with high survival rates, high risk neuroblastoma (HRNB) patients have less than a 50 percent chance of survival. Due to this disparity HRNB patients undergo intense multimodal treatment, while researchers attempt to find the best variants and combinations of treatments that will improve this statistic. One factor of interest in treatment is the autologous stem cell transplant (ASCT). ASCT have been found to improve event free survival (EFS) when compared to continuing chemotherapy, however overall survival (OS) rates remain unchanged. Similarly, tandem ASCT has improved EFS significantly compared to a single transplant, while OS remains the same. While OS is the standard for measuring the efficacy of oncological treatments, with a cancer as aggressive as HRNB improved EFS is satisfactory. Thus, single transplants should be continued, and tandem transplants should be implemented when indicated.
Keywords
Neuroblastoma; Autologous Stem Cells; Treatment; Stem Cell Transplant; Tandem
Introduction
What is Neuroblastoma?
Neuroblasts are immature nerve cells that under normal conditions mature into nerve cells or adrenal medulla cells. When nerve cells do not mature properly, they can continue to grow forming tumors known as neuroblasts [1]. Most neuroblastomas begin in sympathetic nerve ganglia in the abdomen, and about half of those from the adrenal glands. The remaining neuroblastomas typically arise from the sympathetic ganglia near the spine, pelvis, chest, or neck [2]. Every year 800 children in the United States aged zero to fourteen are diagnosed with neuroblastoma making it the third most common form of childhood cancer [1].
Causes
While there is no definitive cause for neuroblastomas several risk factors have been identified. Risk factors can influence the development of cancer, but do not directly cause it [3]. Neuroblastomas occur more often in boys than girls. African and Native American patients are also more likely to have more aggressive disease with lesser survival rates for reasons unknown [4]. So far, no environmental factors have been found to be risk factors for neuroblastomas [3]. In 1-2% of all diagnoses of neuroblastoma there is a family history of the disease, and it is developed due to inherited gene changes [2,3]. In most cases with family history, there are germline mutations in the anaplastic lymphoma kinase (ALK) gene (3). In a few cases with a family history there are changes in the paired-like homeobox (PHOX) 2B gene, which helps the maturation of nerve cells [2]. Neuroblastomas have also been seen in patients with congenital central hypoventilation syndrome (CCHS), a unique disorder of breathing control associated with Hirschsprung disease (HSCR). CCHS results from germline mutations, which may be passed directly from parent to child [1,5]. Majority of neuroblastomas are not due to inherited changes. They are the result of gene changes that happen at some point in the child's development without any known cause, and often the exact gene change is unknown [2].
Staging
There are two systems to stage neuroblastomas. The International Neuroblastoma Staging System (INSS) uses results from the surgery to remove a child's tumor instead of imaging tests to stage the cancer (Table 1). Because of this it cannot help doctors stage a tumor before treatment and is not useful for patients who do not need or cannot have surgery [6,10]. The INSS was developed in 1986 and used for years however, worldwide the factors to determine stages varied making it difficult to compare results from clinical trials. To solve this problem the International Neuroblastoma Risk Group Staging System (INRGSS) was developed (Table 2). This uses results from imaging tests to help decide a stage before treatment begins and creates uniformity of neuroblastoma staging throughout the world [6,7].
Stage 1 |
Localized tumor with complete surgical excision, representative ipsilateral lymph nodes negative for tumor microscopically |
Stage 2A |
Localized tumor with incomplete surgical excision, representative ipsilateral lymph nodes negative for tumor microscopically |
Stage 2B |
Localized tumor, may or may not have had complete surgical excision, representative ipsilateral lymph nodes positive for cancer |
Stage 3 |
Tumor cannot be removed surgically, one of the following is true: tumor has crossed midline of body, tumor has spread to other ipsilateral lymph nodes across midline of body, cancer is in midline of body and is growing bilaterally |
Stage 4 |
Primary tumor has spread to distant lymph nodes, skin, liver, bones, or bone marrow, except for those listed in Stage 4S |
Stage 4S |
Children less than 1, Localized primary tumor (as defined for stage 1, 2A or 2B), with dissemination limited to skin, liver, and/or bone marrow (bone marrow with <10% tumor cell involvement) |
Table 1: International Neuroblastoma Staging System (INSS) criteria [3,6,7,10].
Stage L1 |
Localized tumor, no image defined risk factors |
Stage L2 |
Localized tumor, with at least one image defined risk factor |
Stage M |
Tumor has metastasized, excluding stage MS |
Stage MS |
Tumor has spread to only the skin, liver, and/or bone marrow (less than 10% bone marrow involvement) in patients younger than 18 months |
Table 2: International Neuroblastoma Risk Group Staging System (INRGSS) criteria [3,6,7,10].
Classification
Neuroblastomas are classified as very low, low, intermediate, or high risk. There are numerous deciding factors to determine the classification of a patient's neuroblastoma that include age at diagnosis, stage of disease, histologic category, intermixed versus ganglioneuroblastoma or nodular versus neuroblastoma, MYCN gene status, Chromosome 11q status, and Tumor cell ploidy [1].
What is high risk neuroblastoma?
Non-high-risk patients are typically those without MYCN amplification of the tumor and children under the age of 18 months with metastatic disease. MYCN is an oncogene, or a gene that helps regulate cell growth. When this gene is amplified the neuroblastomas grow more rapidly and the prognosis is worse [6]. Prognosis for non-high-risk patients is very favorable, with a five-year event free survival rate of greater than 95% for low risk and 90-95% for intermediate risk patients [1,8]. Treatment for these cases varies and is as noninvasive as possible. It can include simple observation of the tumor which may regress on its own, surgical removal of the tumor, and chemotherapy when needed [8,9].
High risk neuroblastomas have much less favorable outcomes. The five-year survival rate of children categorized as high risk is less than 50% [1]. High risk neuroblastomas include stage L1 and L2 with MYCN amplification. Any patients with metastatic disease older than 18 months are considered high risk irrespective of MYCN amplification [7]. Less than 18-month-old patients with Stage M tumors with MYCN amplification are considered high risk. Finally, Stage MS in children younger than 18 months with 11q aberration or MYCN amplification are high risk [2]. This is because tumors that are missing part of the 11q chromosome are more likely to spread rapidly linking it to less favorable outcomes [6].This disparity seen between high risk and low or intermediate risk has been the reason for extensive research and multifaceted approaches for treatment of high-risk neuroblastoma.
Treatment
Treatment for high-risk neuroblastoma is extensive due to its aggressive nature. Despite evolving treatment options, it remains difficult to treat with poor outcomes. The current treatment standards include induction, local control, consolidation, and maintenance phases.
Induction
Induction is the first step in treatment of high-risk neuroblastoma and is critical for determining prognostic indicators. Radiolabeled metaiodobenzylguanidine (mIBG) is a highly sensitive and specific marker for detecting neuroblastoma. A semiquantitative mIBG score (Curie score [CS]) is found by measuring mIBG uptake by the neuroblastoma before and after induction treatment. The Curie score allows early indications of prognosis and measures the success of induction [11]. Specific induction regimens vary but typically include 5-8 cycles of intensive chemotherapy including platinum, alkylating, and topoisomerase agents [7]. North American induction regimens include vincristine, doxorubicin, cyclophosphamide, cisplatin, and etoposide. In addition, the Children’s Oncology Group (COG) trials have incorporated topotecan during the first two cycles of induction. The Society of Pediatric Oncology Europe Neuroblastoma Group (SIOPEN) has utilized a rapid COJEC regimen that gives repeated cycles with compression of the recovery interval leading to fourteen-day cycles. Rapid COJEC gives eight total cycles utilizing combinations of vincristine, carboplatin, etoposide, cyclophosphamide, and cisplatin [12,13]. A cochrane review showed no difference in treatment response and inconclusive data about toxicity differences between COG and COJEC protocols [14]. It is during this phase of treatment that stem cells are collected to be used later in the stem cell transplant(s). Autologous stem cell transplants are typically used to treat high risk neuroblastoma (HRNB). Hematopoietic stem cells are collected from the patient's peripheral blood or bone marrow. The cells are then frozen until it is time for the transplant. The goal is to use other treatments to destroy the cancer cells in the body including in the blood and bone marrow. Later when they undergo a stem cell transplant, the hematopoietic stem cells will engraft, or begin to make new blood cells, and turn into healthy, blood-producing tissue in 8 days to 3 weeks. In an autologous stem cell transplant, there is little risk of rejection because the stem cells are your own [15]. In COG protocols stem cells were harvested post cycle 2 of induction and in rapid COJEC stem cells were harvested at the end of the eight induction cycles [13]. As it is not uncommon for patients to have residual bone marrow disease at the time of stem cell collection, the COG conducted a study analyzing the difference between purging the stem cells prior to infusion. The study showed no significant difference in survival (five-year event free survival (EFS) 40% vs. 36%, p = 0.77; five-year overall survival (OS) was 50% vs. 51%, p = 0.81) between purged and unpurged stem cells, so it is not protocol to purge stem cells [16].
Local Control
Typically, local control via surgery is performed towards the end of the induction phase. This is in hopes to shrink the tumor(s) as much as possible before resection [13]. Resection was important in patients with metastasized tumors who responded poorly to induction therapy, but the extent of it was not. This was supported by a COG study that showed in stage 4 tumors, the five-year EFS rate was 30% for patients who achieved complete resection compared with 25% (p= 0.1010) for those without complete resection [17]. This was supported by an additional retrospective analysis of HRNB patients with metastasized tumors that showed no difference in the EFS, or OS based on the extent of resection [18]. However, the extent of resection in children with localized high-risk tumors is important. A retrospective analysis of patients from the German neuroblastoma trial NB97 illustrated that of those children with localized high-risk tumors, those who underwent complete resection had superior EFS and OS compared with patients who had gross total resections, incomplete surgery, or biopsy only [19]. Therefore, in patients with metastatic high-risk disease the goal should be at least partial resection, and complete resection should not occur at the expense of the patient as it shows no significant differences. In patients with localized high-risk disease, complete resection should be the goal. As always with surgery, surgical morbidity, tumor location, and other factors should be carefully considered.
Consolidation
After surgery (when possible), the consolidation phase begins. This includes myeloablative chemotherapy followed by an autologous stem cell transplant. After recovery from the stem cell transplant radiation therapy occurs. Myeloablative, or high dose chemotherapy prior to a stem cell transplant is currently the standard of care. A COG study showed significant improvement in EFS (three-year EFS 34% vs. 22% p = 0.034) for those who underwent high dose chemotherapy with ASCT compared to those who completed continuation chemotherapy. There was, however, no difference in OS (three-year OS 43% ASCT vs. 44% p = 0.87) [20,21]. Additional studies have also suggested that the benefit of myeloablative therapy is minimal in the setting of current treatment regimens. In a retrospective non-randomized study of results at the Memorial Sloan Kettering Cancer Center (MSKCC) patients with high-risk neuroblastoma treated with or without myeloablative therapy had similar survival rates [26]. These mixed results suggest that the optimal consolidation regimen for high-risk neuroblastoma has yet to be determined [7]. After recovery from the ASCT radiation therapy takes place. Radiation is typically applied to the primary tumor site as well as metastatic sites. The current typical amount of radiation used is 21Gy, but studies are ongoing searching for the most effective, but least harmful dose [22,23].
Maintenance
The final stage of treatment for HRNB is the post consolidation or maintenance stage. The goal is to treat any residual disease that remains despite the induction and consolidation regimens in hopes of decreasing the rate of relapse [13]. There have been numerous studies attempting to find the most successful methods for post consolidation therapy. One of the most common treatments is the use of isotretinoin (13-cis-retinoic acid), a synthetic retinoid that decreases division and induces differentiation in neuroblastoma cells [7]. In the COG CCG-3891 study patients were randomly assigned to receive either six cycles of isotretinoin or no further therapy after consolidation. The 3-year EFS rate was significantly better among the patients who were assigned to receive isotretinoin compared to the rate of patients who did not [20,21]. Building off the success of that trial, the COG completed a randomized trial evaluating the standard therapy of isotretinoin or immunotherapy with isotretinoin and ch14.18 in combination with alternating granulocyte-macrophage colony-stimulating factor (GM-CSF) and Interleukin-2 (IL- 2). Ch14.18 is a monoclonal antibody that targets GD2, a cell marker found on the surface of neuroblastoma cells [7]. The patients randomized to the immunotherapy arm showed improved survival compared to isotretinoin alone (two-year EFS 66% vs. 46% p = 0.01, and two-year OS 86% vs. 75% p = 0.02). The drawback to immunotherapy with ch14.18 is the significant side effects associated, including the possibility of allergic reaction, fever, or capillary leak syndrome [24]. There have also been trials testing different antibodies directed against the GD2 ganglioside [7]. Another option for decreasing relapse rates is using difluoromethylornithine (DMFO). DMFO targets an irreversible inhibitor of ornithine decarboxylase, the rate limiting enzyme in polyamine synthesis and whose gene is a known target of MYCN [25]. These are just a few of the options for post consolidation therapy being explored. Because HRNB has such a low survival rate and high chances of relapse, many different avenues of treatment are being pursued to increase patients' chances of survival.
Stem Cell Transplant or Continuing Chemotherapy
While it is still unknown which aspect(s) of treatment for HRNB have the greatest effect on survival, the stem cell transplant is a factor of interest. Myeloablative chemotherapy followed by an autologous stem cell transplant is currently the standard of care. However, one study suggests that ASCT may not be needed to improve outcomes when anti-GD2 immunotherapy and isotretinoin is used for consolidation [26]. This study was conducted at the Memorial Sloan Kettering Cancer Center where the HRNB patients are treated with a slightly altered standard of care. This includes induction chemotherapy, consolidation comprised of anti- GD2 immunotherapy and isotretinoin, localized radiotherapy, and GM-CSF [26]. Treatment at this center does not include is myeloablative therapy with ASCT. The use of ASCT was discontinued in 2003 because those at MSKCC believed that disease control could be obtained without it by focusing on dose intensive induction, more potent anti-GD2 immunotherapy, adding GM-CSF [27], and the addition of isotretinoin [24].Between 2003 and 2013 two groups emerged at the treatment center both treated during the same period and whose consolidative therapy, aside from ASCT, was identical (28). Results were analyzed biostatistically to determine if ASCT improved prognosis. Of the 170 patients were enrolled in this study, 60 were treated following ASCT and 110 were treated following traditional chemotherapy. The myeloablative regimens of the patients who received a stem cell transplant varied including carboplatin-etoposide-melphalan (n = 38), or other myeloablative regimens in single (n = 11) or tandem (n = 11) transplant programs using busulfan, cyclophosphamide, melphalan, and thiotepa [28].
Five-year event free survival rates for the ASCT and the non ASCT patients were 65% and 51% respectively, while both groups had the same five-year overall survival at 76% (Figure 1). The major side effects observed both occurred in ASCT patients. One patient developed acute leukemia 12 months after diagnoses and their NB subsequently relapsed and the other patient died from pulmonary fibrosis 78 months from the NB diagnoses. Since patients who received ASCT underwent that additional step in treatment, the time from their first chemotherapy to when consolidation began was longer than those without ASCT. Subset analysis determined that for patients receiving an ASCT time from 1st chemotherapy to 3F8 and time from ASCT to 3F8 were not significant for EFS. In contrast these variables were found to be significant for those who did not receive the ASCT. Based on these subset analyses, it was concluded that the univariate effect of the improved EFS seen in patients who received an ASCT was most likely a result of a longer time from 1st chemotherapy or longer time from ASCT rather than the transplant improving survival [26].
Figure 1: The study conducted by the MSKCC compared EFS and OS for HRNB patients who underwent ASCT and those who did not between 2003 and 2013. (A) A trend was seen toward better event-free survival for those who received the transplant, however this difference was not statistically significant (p = 0.128). (B) Virtually identical overall survival was seen following transplant or chemotherapy, with no significant difference (p = 0.975) [26].
Ultimately the five-year EFS was similar, and the five-year OS was identical for patients whether they received an ASCT or just traditional chemotherapy. In the multivariate analysis, ASCT was not significantly prognostic for either EFS or OS. This study provides unique insight as most studies on HRNB treatment since 2000 have all included ASCT, allowing this study to assess if ASCT should be standard in treatment. Additionally, the two groups had similar clinical and biological characteristics, including MYCN, contemporary induction, and pre-minimal residual disease (MRD)-positivity. This study could suggest that there is a need for reevaluation of stem cell transplants in the treatment of HRNB. This study concludes that ASCT does not have a significant effect on improving EFS or OS when local RT, anti-GD2 mAbs, and isotretinoin are used for consolidation after dose-intensive induction chemotherapy and suggests reevaluating the use of ASCT in treating HRNB [26].
However, in this study ASCT patients were referred to the MSKCC after completing the transplant. The exact myeloablative regimen varied and the number of cells transplanted is not reported both of which could affect the efficacy. Additionally, this trial was not a randomized trial which would be needed to definitively determine if these results accurately represent the wider population of those with HRNB.
Continued Evaluation of Stem Cell Transplant or Continued Chemotherapy
Decades of studies have compared the outcomes of HRNB patients when receiving ASCT or just chemotherapy. However, many of these studies such as the one conducted by the MSKCC were retrospective or not randomized which introduces selection bias. The largest randomized study was conducted by the Children's Cancer Group in the 3891 study. Between 1991 and 1996 the study compared continuing chemotherapy with myeloablative chemotherapy and ASCT in 539 patients. All patients received five cycles of induction with cisplatin, doxorubicin, etoposide, and cyclophosphamide plus surgery and received radiotherapy for residual local and metastatic disease. Patients in the ASCT group received carboplatin and etoposide as continuous infusions over 96 hours; melphalan (140 mg/m2 on day −7 and 70 mg/m2 on day −6); and total-body irradiation (3.33 Gy daily on days −3, −2, and −1) followed by infusion of immunomagnetically purged bone marrow and granulocyte-macrophage colony-stimulating factor. Patients in the continuing chemotherapy (CC) group received three cycles of cisplatin, etoposide, and doxorubicin, as continuous infusions over 96 hours with bolus ifosfamide (2,500 mg/m2 on days 0 to 3) and GM-CSF [21]. A second random assignment occurred after these consolidation treatments to either receive biologic therapy with isotretinoin or not. Of the 539 eligible patients, 190 were randomly assigned to CC, of those 150 received chemotherapy according to protocol. 189 patients were assigned to ABMT and of those assigned to ABMT 129 received ABMT according to protocol [21]. The assigned treatment was not received in 100 patients. Fifty-two patients had progressive disease (PD) before they could receive assigned therapy (28 in CC group; 24 in ABMT). Two patients died after random assignment but before they could receive the assigned treatment. The remaining 46 patients did not receive assigned therapy because of physician or parent decisions [21].
The five-year EFS for patients who randomly received AMBT was significantly higher than those who received continuing chemotherapy. The EFS rates were 30% ± 4% and 19% ± 3% respectively. The five-year OS rates for AMBT and continuing chemotherapy were 39% ± 4% and 30% ± 4%, respectively (Figure 2). By using the log-rank test to measure the overall difference between the curves, the OS for patients in the ABMT group was not statistically significantly higher than for the patients in the continuing chemotherapy group (by log-rank test; P = .08) [29]. 130 patients were assigned to 13-cis-retinoic acid (cis-RA) and 128 patients were randomly assigned to not receive cis-RA [21]. There was improvement in EFS for those who received cis-RA versus no cis-RA, though it was not statistically significant. 5-year EFS rates from time of random assignment were 42% ± 5% versus 31% ± 5%, respectively (P = .1219) [21].The OS also improved for those who received cis-RA but it was not statistically significantly higher than that of the patients who received no cis-RA. The 5-year OS rates from the time of random assignment were 50% ± 5% v 39% ± 5% respectively and it was not significantly different (P = .10) (Figure 2) [29].
Figure 2: The Children’s Cancer Group 3891 study showed significant improvement in EFS but not OS for patients who received an ABMT and patients in the second random assignment who received cis-RA. (A) Event-free survival for patients who participated in both the first and second random assignments (autologous bone marrow transplantation and cis-RA (n = 50) versus continuing chemotherapy and no cis-RA (n = 53) was statistically significant (P = .0038). Overall survival for patients who participated in both the first and second random assignments did not show a statistically significant improvement (P = .0540) [21].
This large, randomized study had a follow up period of eight years to ensure most possible side effects or events would be observed. More than 91% of the expected events are already observed, so the results of this analysis are stable. This shows the unequivocal benefit of myeloablative chemotherapy with AMBT for high-risk neuroblastomas. The significantly improved 5-year EFS provided support for the continuing use of ASCT in the standard of care for HRNB. Additional randomized trials show similar improvements in EFS for patients who received ASCT versus just chemotherapy [30,31]. The 5-year EFS rate for all patients on CCG- 3891 was 30% compared with 38% for the European Neuroblastoma Study Group 1 [31]. There is however no statistically significant improvement in the OS seen in this study or the other randomized studies [28,29].
The treatment related toxicities of patients studied here included four who had second malignant neoplasms. Two of these occurred in the AMBT and CC groups each. Ultimately 297 patients died from progressive disease, 57 from treatment, and 11 for reasons unknown. Of those who died in treatment 22 were treated with just AMBT and 22 with just CC [21]. The improved EFS with ASCT seen here contradicts the results found by the MSKCC. The improved EFS has been seen in numerous randomized studies and the MSKCC was not a randomized trial. In addition, the induction regimens and stem cell transplant protocols varied in the MSKCC study. This suggests that their findings were not an accurate depiction of the wider population of HRNB. While the ASCT has not been found to improve OS, the EFS rates are significantly higher allowing it to remain a part of the standard of care for HRNB.
Early study of tandem stem cell transplantation
An additional consideration for HRNB stem cell transplants is if a single or tandem transplantation should occur. A nonrandomized retrospective study analyzed cases between November 1996 and November 2008 of HRNB patients who underwent single or tandem transplants. 86 patients underwent treatment for HRNB at Children's Healthcare of Atlanta and another 12 were referred for stem cell transplants from other institutions. 14 patients were excluded from analysis in this study (seven who could not proceed with a transplant, four who relapsed, and three who received 131I‐MIBG as a part of another study) leaving 84 patients for review. All patients received a minimum of five cycles of induction chemotherapy, however due to the retrospective nature of this study, the chemotherapeutic regimens varied as new regimens were found to have better outcomes throughout the duration of this analysis. Following these 28 patients underwent a single ASCT and 56 underwent a tandem ASCT. The median number of cells infused for patients who underwent a single HDC/SCR was 6.05 × 106/kg. The median doses of CD34+ cells infused for patients who underwent tandem HDC/SCR, were 5.18 and 5.29 × 106/kg for the first and second transplant, respectively [32]. Survival times were measured from the infusion day of the single transplant or the second of tandem transplants to the day of relapse, progression, death, or the last follow‐up date for patients in remission. EFS and overall survival (OS) rates were estimated using the Kaplan–Meier method and survival functions were compared using the log‐rank test. The 4-year EFS for patients who received a single HDC/SCR was 27 ± 9%, while EFS for patients who underwent tandem HDC/SCR was 59 ± 7% (log‐rank P‐value = 0.01) (Figure 3A). The 4‐year OS for patients who received a single HDC/SCR was 45 ± 11%, and for patients who underwent tandem HDC/SCR 71 ± 9% (log‐rank P‐value = 0.06) (Figure 3B) [32]. The improved EFS suggests that tandem ASCT could be indicated in HRNB. However, this was a retrospective study with non-random assignment to experimental groups creating a major source of bias. Additionally, the small number of cases studied is a source of bias, and to apply this to the majority of HRNB a randomized trial needs to occur. Furthermore, OS was not significantly improved by tandem ASCT [32].
Another factor to consider with tandem SCT is toxicity. During this study three children died following transplant. One died from gastrointestinal bleeding following a single transplant and two died from veno‐occlusive disease of the liver after receiving tandem transplants. None of the patients who underwent a single transplant developed VOD while 17% of those that received a tandem transplant developed it (it was reversible in 7/9 of them). These additional toxicities were expected, and mostly reversible however there is still limited knowledge on the long-term effects of ASCT, specifically tandem [32]. This initial study was promising, but the lack of randomization, changing induction regimens, and small sample size made it important to conduct further studies to confirm or deny the findings that tandem ASCT increases EFS. This study led to the development of the Children's Oncology Group (COG) trial ANBL0532, which randomized patients to receive single or tandem SCR following induction chemotherapy and surgery.
COG Study of Tandem Stem Cell Transplants
Following previous non-random or small-scale studies comparing tandem and single transplants [32-34], COG conducted a randomized clinical trial November 2007 to February 2012 at 142 Children’s Oncology Group centers in the United States, Canada, Switzerland, Australia, and New Zealand. The goal of this trial was to determine if tandem ASCT improved EFS compared to single ASCT for treatment of HRNB. To enroll in this study patients were required to be 30 years or younger; have adequate kidney, cardiac, and liver function; and be anticipated to have the ability to tolerate PBSC collection (35). They were also required to have HRNB as described by COG criteria including any INSS stage with MYCN amplification, and metastatic stage 3 or 4 [36]. Of the 652 patients enrolled in this trial 297 did not undergo randomization because they were not randomly assigned (n = 27), ineligible for randomization (n = 62), had no therapy (n = 1), or because of physician/parent preference (n = 207). 176 patients were randomly assigned to the tandem transplant group and 179 patients were randomly assigned to the single transplant group.
Induction in this trial included 2 cycles of topotecan/cyclophosphamide, after which patients underwent PBSC collection followed by 4 alternating cycles of cisplatin/etoposide and doxorubicin/cyclophosphamide/vincristine [37]. To move onto the consolidation phase there must have been no disease progression; no uncontrolled infection; recovery from induction therapy toxicity; sufficient PBSC level (≥4×106 CD34+ cells/kg); and adequate kidney, cardiac, and liver function (35). After induction, patients with better response or prognosis were nonrandomly given one stem cell transplant to minimize toxicity. In the consolidation phase, patients randomized to a single transplant received carboplatin, etoposide, and melphalan [37]. Patients randomized to tandem transplants received cyclophosphamide/thiotepa followed by dose-reduced carboplatin, etoposide, and melphalan 6 to 10 weeks later. For a second transplant to occur, there had to be no evidence of disease progression; available PBSC; resolution of acute toxicity from the first transplant; adequate cardiac, kidney, hematopoietic, and hepatic function; no uncontrolled infection; and no history of moderate or severe sinusoidal obstruction syndrome during the first transplant [35]. Both single and tandem transplant patients received at least 1×106 CD34+ cells/kg during their transplant. Following the transplant(s) patients underwent radiotherapy often at the MIBG sites detected after induction therapy. Finally, in the post consolidation treatment phase patients took 2 oral isotretinoin for 14 days of each month for 6 months. Per protocol, patients were strongly encouraged to enroll in 1 of 2 Children’s Oncology Group trials (ANBL0032 or ANBL0931), which evaluated anti disialoganglioside (GD2) chimeric antibody and cytokines immunotherapy [38,24].
The most prevalent side effects across both groups were mucosal (12.9%) and infectious (17.4%), with rare occurrence of sinusoidal obstructive syndrome (3.6%). There were 17 deaths 7 in induction and 10 in consolidation. In induction deaths were due to infection (3), sinusoidal obstruction syndrome [1], surgical complications [2], and cardiac failure [1]. Death during consolidation occurred in 7 patients in the single transplant group and 2 in the tandem transplant group. In the single transplant group deaths were caused by sinusoidal obstructive syndrome [4], sepsis [2], multiorgan failure [1], and one from symptoms suggestive of transplant-related microangiopathy. In the tandem transplant group, 1 death was caused by symptoms suggestive of transplant-related microangiopathy and 1 from respiratory failure following the initial transplant [35].
Figure 4: Results of the Children's Oncology Group randomized study comparing single and tandem ASCT. P values were calculated using a 1-sided log-rank test for the primary analysis and a 2-sided log-rank test for the post hoc analyses. (A)The EFS for patients in the tandem transplant group was significantly higher than EFS of patients randomized to the single transplant group (P=.006). (B) Three-year OS was not significantly different for patients in the tandem transplant group compared with the single transplant group (P = .25) (C) Three-year EFS from the time of initiating immunotherapy was higher in the tandem transplant group compared with the single transplant group (P = .004). (D) Three-year OS from the time of initiating immunotherapy was higher in the tandem transplant group compared with the single transplant group (P = .04) [35].
The most prevalent side effects across both groups were mucosal (12.9%) and infectious (17.4%), with rare occurrence of sinusoidal obstructive syndrome (3.6%). There were 17 deaths 7 in induction and 10 in consolidation. In induction deaths were due to infection (3), sinusoidal obstruction syndrome [1], surgical complications [2], and cardiac failure [1]. Death during consolidation occurred in 7 patients in the single transplant group and 2 in the tandem transplant group. In the single transplant group deaths were caused by sinusoidal obstructive syndrome [4], sepsis [2], multiorgan failure [1], and one from symptoms suggestive of transplant-related microangiopathy. In the tandem transplant group, 1 death was caused by symptoms suggestive of transplant-related microangiopathy and 1 from respiratory failure following the initial transplant [35].
The primary goal of this trial was to compare single versus tandem EFS using a one-sided log rank test. EFS curves were generated using the Kaplan-Meier Method [39]. The OS rates were compared using a two-sided log-rank test. The three-year EFS of this study was 61.6% and 48.4% for tandem and single transplants respectively [35]. EFS from the time of randomization was significantly higher (1-sided log-rank P = .006) for the group who underwent a tandem transplant (Figure 4A). The three-year OS was 74.1% and 69.1% for tandem and single transplants respectively. This difference was not statistically significant (P = .25) (Figure 4B). After consolidation therapy 250 of the 355 patients enrolled in COG trial ANBL0032 or ANBL0931. Three-year EFS and OS from the time of initiating post consolidation immunotherapy of patients in these trials were statistically significant and higher in the tandem transplant group compared with the single transplant group (Figure 4C and 4D) [35]. The randomized trial concluded that tandem ASCT greatly improved the three-year EFS, but with no effect on the OS.
The limitations of this study include the number of patients that were not randomized, introducing the possibility of selection bias. Additionally, patients who underwent tandem transplants subsequently had longer and more expensive hospital stays. These findings are only able to be applied to the specific induction regimen used. It is not known if the same improve in EFS would be seen within other newer induction regimens.
Conclusion
When studying the efficacy of treatments for HRNB the two main factors compared are the EFS and OS. Currently, overall survival is seen as the gold standard for evaluating the outcome of any treatment in oncologic clinical trials. This is because it is an unbiased defined end point with great clinical relevance and accurately describes the efficacy of treatments [40]. As discussed, randomized trials have found that patients who receive one ASCT have a higher EFS and the same OS as those who undergo continuing chemotherapy [21,30,31]. Additionally, patients have had improved EFS when receiving a tandem ASCT compared to just one, but still the OS remains not statistically different [32, 35]. Although no improvement was found in OS for these varying methods of treatment, significant EFS improvements were seen. Although EFS is not the gold standard for oncology trials, when a cancer is as aggressive as HRNB physicians must take any improvement as a benefit. Since ASCT improved the EFS, they are still in the standard of treatment for HRNB because any improvement is better than none. It is the hope of researchers that as the multimodal treatment of HRNB continues to expand, the right combination of treatments will continue to increase EFS and OS.
Another factor to consider with HRNB is the probability of relapse. Despite the advances being made in treatment many HRNB patients will respond poorly to treatment or will experience disease recurrence [7]. In one study the five-year OS was only 20% after the first event of relapse [41]. Treatment for relapsed HRNB will be similar, but more aggressive than the original treatment. As a result, the associated toxicities will be much greater and possibly detrimental to the child.
Toxicities associated with HRNB vary based on the treatment received. When considering if a patient should receive a single or tandem transplant the physicians must consider the toxicities associated with two transplants versus one and if the patient can survive that [35]. The main consideration for all treatment is minimizing the toxicities while maximizing the child's chance for survival. In a retrospective study of patients at the Dana-Farber Cancer Institute/Boston Children’s Hospital from September 1994 to October 2007, the lasting effects of HRNB treatment were recorded [42]. The effects included endocrine changes in 24 patients with 11/24 developing pre-diabetes. Compensated hyperthyroidism was also found in 49% of the patients. Male and female patients saw decreased fertility with 75% of the females developing premature ovarian insufficiency. Non endocrine related effects most patients experienced was hearing loss (73%) and dental disease (51%) [42]. Additionally, while rare second malignant neoplasms are a potential side effect occurring in 2 to 7% of patients [43,44]. Treatment of HRNB typically includes alkylating agents, topoisomerase II inhibitors, platinum compounds, and radiotherapy all of which are known risk factors for secondary malignancies [44].
As previously discussed, there are inherent risks associated with stem cell transplantation, whether it is single or tandem. The most common effects from a transplant seen in the COG study were mucosal and infectious, with rare occurrence of sinusoidal obstructive syndrome [35]. Additionally, the retrospective study conducted by Children's Healthcare of Atlanta found that some children who received tandem transplants developed VOD of the liver while none of those who received a single transplant did [32]. The toxicity is expected to be more severe in tandem transplants, as those patients undergo a whole additional ‘round’ of treatment. Overall, not enough is known about the long-term effects single or tandem ASCT will have on children with HRNB. Physicians should carefully weigh the need for a tandem transplant based on how the child recovered from the first, if there has been response to treatment, and disease progression while weighing the benefit it could have on extending their life over the potential harm. Ultimately, a single ASCT remains the standard of care for HRNB as it shows improved EFS and has for decades [21,30,31]. Tandem ASCT is used less regularly; however this could change if more studies are conducted to prove its benefit and learn more about toxicity associated with it.
References
- https://www.cancer.net/cancer-types/neuroblastoma-childhood/statistics
- https://www.cancer.org/cancer/neuroblastoma/about/what-is-neuroblastoma.html
- https://www.cancer.net/cancer-types/neuroblastoma-childhood/risk-factors
- Henderson TO, Bhatia S, Pinto N, London WB, McGrady P, et al. (2011) Racial and ethnic disparities in risk and survival in children with neuroblastoma: a Children's Oncology Group study. J Clin Oncol. 29(1):76-82.
- Rohrer T, Trachsel D, Engelcke G, Hammer J. (2002) Congenital central hypoventilation syndrome associated with Hirschsprung's disease and neuroblastoma: case of multiple neurocristopathies. Pediatric pulmonol. 33(1):71-6.
- https://www.cancer.org/cancer/neuroblastoma/detection-diagnosis-staging/staging.html
- Whittle SB, Smith V, Doherty E, Zhao S, McCarty S, et al (2017) Overview and recent advances in the treatment of neuroblastoma. Expert Rev Anticancer Ther. 17(4):369-86.
- Baker DL, Schmidt ML, Cohn SL, Maris JM, Buxton A, et al. (2010) Outcome after reduced chemotherapy for intermediate-risk neuroblastoma. N Engl J Med. 363:1313-23.
- Strother DR, London WB, Schmidt ML, Brodeur GM, Shimada H, et al. (2012) Outcome after surgery alone or with restricted use of chemotherapy for patients with low-risk neuroblastoma: results of Children's Oncology Group study P9641. J Clin Oncol. 30(15):1842-48.
- Brodeur GM, Seeger RC, Barrett A, Berthold F, Castleberry RP, et al. (1988). International criteria for diagnosis, staging, and response to treatment in patients with neuroblastoma. J Clin Oncol. 6(12):1874-81.
- Yanik GA, Parisi MT, Shulkin BL, Naranjo A, Kreissman SG, et al. (2013). Semiquantitative mIBG scoring as a prognostic indicator in patients with stage 4 neuroblastoma: a report from the Children's oncology group. J Nucl Med. 54(4):541-48.
- Pearson AD, Pinkerton CR, Lewis IJ, Imeson J, Ellershaw C, et al. (2008) High-dose rapid and standard induction chemotherapy for patients aged over 1 year with stage 4 neuroblastoma: a randomised trial. The Lancet. Oncol. 9(3):247-56.
- Smith V, Foster J. (2018) High-Risk Neuroblastoma Treatment Review. Children. 5(9):114.
- Peinemann F, Kahangire DA, Van Dalen EC, Berthold F. (2015) Rapid COJEC versus standard induction therapies for high-risk neuroblastoma. Cochrane Database Syst Rev. 60:1135-51.
- https://www.cancer.net/cancer-types/neuroblastoma-childhood/types-treatment
- Kreissman SG, Seeger RC, Matthay KK, London WB, Sposto R. et al. (2013) Purged versus non-purged peripheral blood stem-cell transplantation for high-risk neuroblastoma (COG A3973): a randomised phase 3 trial. The Lancet Oncol. 14(10):999-1008.
- Adkins ES, Sawin R, Gerbing RB, London WB, Matthay KK, et al. (2004) Efficacy of complete resection for high-risk neuroblastoma: a Children's Cancer Group study. J pediatric surg. 39(6):931-36.
- Du L, Liu L, Zhang C, Cai W, Wu Y, et al. (2014) Role of surgery in the treatment of patients with high-risk neuroblastoma who have a poor response to induction chemotherapy. Journal of pediatric surg. 49(4):528-33.
- Fischer J, Pohl A, Volland R, Hero B, Dübbers M, et al. (2017) Complete surgical resection improves outcome in INRG high-risk patients with localized neuroblastoma older than 18 months. BMC cancer. 17(1):520.
- Matthay KK, Villablanca JG, Seeger RC, Stram DO, Harris RE, et al. (1999) Treatment of high-risk neuroblastoma with intensive chemotherapy, radiotherapy, autologous bone marrow transplantation, and 13-cis-retinoic acid. Children's Cancer Group. The New England j med. 341(16):1165-73.
- Matthay KK, Reynolds CP, Seeger RC, Shimada H, Adkin ES, et al. (2009) Long-term results for children with high-risk neuroblastoma treated on a randomized trial of myeloablative therapy followed by 13-cis-retinoic acid: a children's oncology group study. Journal of clinical oncol. 27(7):1007-1013.
- Brodeur GM, Pritchard J, Berthold F, Carlsen NL, Castel V, et al. (1994). Revisions of the international criteria for neuroblastoma diagnosis, staging and response to treatment. Prog Clin Biol Res.385:363-69.
- Hill-Kayser C, Tochner Z, Both S, Lustig R, Reilly A, et al. (2013) Proton versus photon radiation therapy for patients with high-risk neuroblastoma: the need for a customized approach. Pediatr blood cancer. 60(10):1606-11.
- Yu AL, Gilman AL, Ozkaynak MF, London WB, Kreissman SG, et al. (2010) Anti-GD2 antibody with GM-CSF, interleukin-2, and isotretinoin for neuroblastoma. N Engl J Med. 363(14):1324-34.
- Geerts D, Koster J, Albert D, Koomoa DL, Feith DJ, et al. (2010). The polyamine metabolism genes ornithine decarboxylase and antizyme 2 predict aggressive behavior in neuroblastomas with and without MYCN amplification. Int j cancer. 126(9):2012-24.
- Kushner BH, Ostrovnaya I, Cheung IY, Kuk D, Modak S, et al. (2016) Lack of survival advantage with autologous stem-cell transplantation in high-risk neuroblastoma consolidated by anti-GD2 immunotherapy and isotretinoin. Oncotarget. 7(4):4155-166.
- Kushner BH, Kramer K, Cheung NK. (2001) Phase II trial of the anti-G(D2) monoclonal antibody 3F8 and granulocyte-macrophage colony-stimulating factor for neuroblastoma. J Clinic Oncol. 19(22):4189-194.
- Panageas KS, Ben-Porat L, Dickler MN, Chapman PB, Schrag D. (2007) When you look matters: the effect of assessment schedule on progression-free survival. J National Cancer Instit. 99(6):428-32.
- https://ascopubs.org/doi/10.1200/JCO.2014.57.0382
- Berthold F, Boos J, Burdach S, Erttmann R, Henze G, et al. (2005) Myeloablative megatherapy with autologous stem-cell rescue versus oral maintenance chemotherapy as consolidation treatment in patients with high-risk neuroblastoma: a randomised controlled trial. Lancet Oncol. 6(9):649-58.
- Pritchard J, Cotterill SJ, Germond SM, Imeson J, de Kraker J, et al. (2005) High dose melphalan in the treatment of advanced neuroblastoma: results of a randomised trial (ENSG‐1) by the European Neuroblastoma Study Group. Pediatr Blood Cancer. 44(4):348-57.
- Qayed M, Chiang KY, Ricketts R, Alazraki A, Tahvildari A, et al. (2012) Tandem stem cell rescue as consolidation therapy for high‐risk neuroblastoma. Pediatr Blood Cancer. 58(3):448-52.
- Seif AE, Naranjo A, Baker DL, Bunin NJ, Kletzel M, et al. (2013) A pilot study of tandem high-dose chemotherapy with stem cell rescue as consolidation for high-risk neuroblastoma: Children's Oncology Group study ANBL00P1. BMT. 48(7):947:52.
- Granger M, Grupp SA, Kletzel M, Kretschmar C, Naranjo A, et al. (2012) Feasibility of a tandem autologous peripheral blood stem cell transplant regimen for high-risk neuroblastoma in a cooperative group setting: a Pediatric Oncology Group study: a report from the Children's Oncology Group. Pediatr Blood Cancer. 59(5):902-07.
- Park JR, Kreissman SG, London WB, Naranjo A, Cohn SL, ET AL. (2019) Effect of Tandem Autologous Stem Cell Transplant vs Single Transplant on Event-Free Survival in Patients with High-Risk Neuroblastoma: A Randomized Clinical Trial. JAMA. 322(8):746-55.
- Irwin MS, Park JR. (2015) Neuroblastoma: paradigm for precision medicine. Pediatr Clin N Am. 62(1):225-56.
- Park JR, Scott JR, Stewart CF, London WB, Naranjo A, et al. (2011) Pilot induction regimen incorporating pharmacokinetically guided topotecan for treatment of newly diagnosed high-risk neuroblastoma: a Children's Oncology Group study. J Clin Oncol. 29(33):4351-57.
- Ozkaynak MF, Gilman AL, London WB, Naranjo A, Diccianni MB, et al. (2018) A Comprehensive Safety Trial of Chimeric Antibody 14.18 With GM-CSF, IL-2, and Isotretinoin in High- Risk Neuroblastoma Patients Following Myeloablative Therapy: Children's Oncology Group Study ANBL0931. Front Immunol. 9:1355.
- Kaplan EL,Meier P. (1958) Nonparametric Estimation from Incomplete Observations. J Am Stat Assoc. 53:457-81.
- Driscoll JJ, Rixe O. (2009) Overall survival: still the gold standard: why overall survival remains the definitive end point in cancer clinical trials. J Cancer. 15(5):401-05.
- London WB, Castel V, Monclair T, Ambros PF, Pearson AD, et al. (2011) Clinical and biologic features predictive of survival after relapse of neuroblastoma: a report from the International Neuroblastoma Risk Group project. J Clin Oncol. 29(24):3286-92.
- Cohen LE, Gordon JH, Popovsky EY, Gunawardene S, Duffey-Lind E, et al. (2014) Late effects in children treated with intensive multimodal therapy for high-risk neuroblastoma: high incidence of endocrine and growth problems. BMT. 49(4):502-8.
- Applebaum MA, Henderson TO, Lee SM, Pinto N, Volchenboum SL, et al. (2015) Second malignancies in patients with neuroblastoma: the effects of risk-based therapy. Pediatr Blood Cancer. 62(1):128-33.
- Federico SM, Allewelt H, Spunt SL, Hudson MM, Wu J, et al. (2015) Subsequent malignant neoplasms in pediatric patients initially diagnosed with neuroblastoma. Pediatr Hematol Oncol J. 37(1):e6-e12.