The Effect of Stem Cells on Kidney Disease
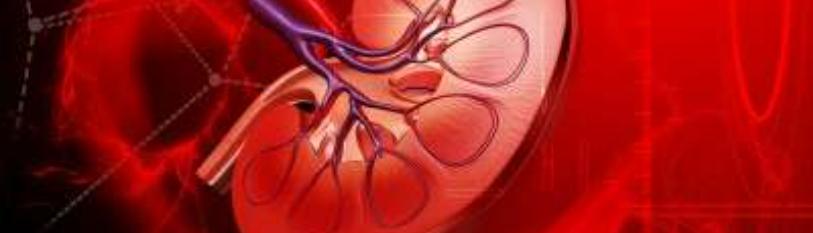
McCrady Andrews1, Mohammed S Inayat2 and Vincent S. Gallicchio1*
1Department of Biological Sciences, College of Science, Clemson University, Clemson, South Carolina, USA, 29636
2Department of Internal Medicine, Division of Hospital Medicine, University of Cincinnati College of Medicine 231 Albert Sabin Way, ML 0535 Cincinnati, OH 45267
*Corresponding author: Vincent S. Gallicchio, Department of Biological Sciences, College of Science, Clemson University, Clemson, South Carolina, USA, 29636
Citation: Andrews MC, Inayat MS, Gallicchio VS. (2024) Stem Cells as Potential Regenerative Treatment for Retinal Degenerative Diseases and Diabetic Retinopathy. .J Stem Cell Res. 5(1):1-26.
Received: December 8, 2023 | Published: January 5, 2024
Copyright© 2024 genesis pub by Andrews MC, et al. CC BY NC-ND 4.0 DEED. This is an open-access article distributed under the terms of the Creative Commons Attribution-NonCommercial-No Derivatives 4.0 International License., This allows others distribute, remix, tweak, and build upon the work, even commercially, as long as they credit the authors for the original creation.
DOI: https://doi.org/10.52793/JSCR.2024.5(1)-54
Abstract
Kidney-related diseases including chronic kidney disease, acute kidney injuries, and end stage kidney disease are global public health matters with mortality and incidence rates increasing in recent years. Acute kidney injury is experienced by one-fifth of all adults and one-third of all children. Approximately 13.6% of Americans are diagnosed with chronic kidney disease. The increased morbidity and poor prognosis place a large burden on health systems in the public. It is estimated that more than $49 billion is spent every year on treatment for patients with chronic kidney disease. The kidneys play an important role in the elimination of toxic metabolites. When the kidney is weakened, toxic substances assemble in the bloodstream and result in biochemically toxic effects in many tissues and organs.
This issue causes additional complications including: anemia, cardiovascular disease, and neurological disorders. End stage kidney disease occurs when chronic kidney disease reaches an advanced stage and kidneys lose their ability to filter. Patients with end stage kidney disease have a high mortality risk accounting for 53% of deaths. At this stage, renal replacement therapies including dialysis or a kidney transplant are required to increase a patient’s life expectancy. Although surgical or pharmaceutical therapies may improve the overall function of the kidney, they cannot improve the regeneration and functional recovery of the tissues near the affected kidney damage.
This issue causes additional complications including: anemia, cardiovascular disease, and neurological disorders. End stage kidney disease occurs when chronic kidney disease reaches an advanced stage and kidneys lose their ability to filter. Patients with end stage kidney disease have a high mortality risk accounting for 53% of deaths. At this stage, renal replacement therapies including dialysis or a kidney transplant are required to increase a patient’s life expectancy. Although surgical or pharmaceutical therapies may improve the overall function of the kidney, they cannot improve the regeneration and functional recovery of the tissues near the affected kidney damage.
Keywords
Kidney disease; Stem cells; Treatment
Abbreviations
ACE: anglotensin converting enzyme; ACR: albumin to creatinine ratio; ADPICD: autosomal dominant polycystic kidney disease; AE: adverse event; AKI: acute kidney injury; AMR: antibody mediated rejection; ARVD: atherosclerotic renovascular disease; AT-MSC: adipose tissue mesenchymal stem cell; BMMS: bone marrow mesenchymal stromal cell; BUN: blood urea nitrogen; CAMR: chronic active antibody-mediated rejection; CBMSC: cord blood mesenchymal stem cell; CKD: chronic kidney disease; CVD: cardiovascular disease; DM: diabetesmellitus; DN: diabeticnephropathy; ELISA: enzyme linked immunoassay; ESRD: end-stage renal disease; EV: extracellular vesicles; GFR: glomerular filtration rate; HUMUSC: human umbilical mesenchymal stem cell; IL: interleukin; KTR: kidney transplant recipients; MDR-INS: multi-drug resistant idiopathic nephrotic syndrome; MSC: human mesenchymal stem cell; MV: microvesicles; NKF-KDOQI:National Kidney Foundation’s Kidney Disease Outcomes Quality Initiative; NSAID: non steroid anti-inflammatory drug; RNA: ribonucleic acid; UCB-MSC: umbilical cord blood mesenchymal stem cell; USC-EXO: urine derived stem cells – exosomes; VEGF: vascular endothelial growth factor.
Introduction
The renal system consists of the urethra, ureters, and kidney. Filtration is the main function of the system as it filters approximately a couple hundred liters of fluid per day from renal blood flow which allows for metabolic waste products, toxins, and excess ion to be excreted while keeping needed substances in the blood. The kidney helps with the regulation of the plasma osmolarity by regulating the amount of electrolytes, water, and solutes in the blood. It ensures long-term balance of the acidity and basicity. It also produces erythropoietin which stimulates the production of red blood cells. Renin is also created for the regulation of blood pressure and converts vitamin D to its active form [1-4].
The kidneys can play a great role in the elimination of metabolites that are toxic, such as uremic toxins. When the purpose of kidney is weakened, these toxic substances assemble in the bloodstream and result in biochemically toxic effects in many tissues and organs, which causes additional complications, including, anemia, cardiovascular disease, and disorders relating to neurology [1-4].
Kidney disease prevalence and mortality rates continue to increase despite medical advances [8-9]. Extracellular matrix that has accumulated causes decreased renal function that leads to CKD. Approximately 13.6% of Americans are diagnosed with chronic kidney disease (CKD) [8-10].
The increased morbidity and poor prognosis place a large burden on health systems in the public, and it is estimated that north of forty-nine billion dollars are spent every year for treating patients with chronic kidney disease [8-9]. AKI has many possible causes, including renal ischemia from decreased blood pressure, inflammation, crush injury, and urinary tract infection or obstruction [11-12]. It is diagnosed based on increased blood urea nitrogen (BUN) or creatinine concentrations or deceased output of urine [11-12].
Common Etiologies of Acute Kidney Injury
Prerenal |
Cardiac Output |
acute myocardial infarction, valve rupture, acute pericarditis, acute myocarditis, drugs exacerbate heart failure (COX inhibitors, CCB, TZD, DPP-41), drugs cause direct heart injury (rheumatologic agents, anthracyclines, tisanes, targeted therapy, anti-Parkinson) |
|
True Hypovolemia |
renal loss (diuretics, osmotic diuresis), extrarenal loss (diarrhea, hemorrhage, burn, third spacing) |
|
Effective Volume |
sepsis, neurogenic shock, anaphylaxis |
|
Intrarenal Vasoconstrictor |
hypercalcemia, hepatorenal syndrome, drugs, (CNIs, NSAID, vasoconstrictors) |
Intrinsic |
Glomerular Injury |
nephrotic (MCD, MPGN, drugs (NSAID, gold, penicillamine)), 213607 nephritic (IRGN, lupus, nephritis, AAV, anti-GBM disease, IgAN, drugs (hydralazine)) |
|
Tubular Injury |
severe prerenal causes, toxins, endogenous: hemolysis, rhabdomyolysis, tumor lysis syndrome) or exogenous (aminoglycoside, contrast, CNIs, acyclovir, lithium, vancomycin)) |
|
Interstitial Injury |
allergy (drug: cephalosporin, penicillin, PPI, NSAID, herbs), infection (bacteria, fungus, virus, leptospirosis), autoimmune (Lupus, anti-TBM disease, AAV) |
|
Vascular Injury |
small caliber (TMA (malignant hypertension, HUS/TTP, DIC), scleroderma renal crisis) large caliber (renal infarction, renal vein thrombosis) |
Postrenal |
Urinary Tract |
benign prostatic hyperplasia, neurogenic bladder, intra-ureter (stones, tumors), extraureter (retroperitoneal fibrosis, intra-abdominal tumors) lesions |
|
Intrarenal |
Crystals (acyclovir, indinavir), stones, tumors, paraproteins (myeloma) |
Table 1: Outlines common etiologies of acute kidney injury [12].
The mortality rate in AKI patients is about fifty percent. The majority of surviving patients recover full renal function. However, some patients develop CKD, requiring further treatment, such as renal transplantation and dialysis [13]. CKD is characterized by a progressive loss of the purpose of the kidney, leading to end-stage renal disease (ESRD) and collage accumulation, due to inflammation, leading to fibrosis [14]. At the ending stage of CKD, irreversible renal function loss is treated with transplantation of the kidney or dialysis. AKI can also result in ESRD, leading to a rise in the risk of CKD or aggravated CKD symptoms [7-15]. CKD is a progressive disease as well, leading to significant morbidity and mortality. Although surgical or pharmaceutical therapies may improve the overall function of the kidney, they cannot improve the regeneration and functional recovery of the tissues near the affected kidney damage. Therefore, there is a need to develop more effective strategies for treating injury to the kidney [11-12].
There is a high variability in CKD that are estimated in glomerular filtration rate (GFR) trajectories. This occurrence can insinuate that there are a large array of heterogenous risk factors potentially contributing to the overall development of CKD. If we wish to attain renoprotection, then these risk factors may end up becoming certain targets of therapy. Certain lifestyle factors such as one’s diet, loss of sleep and exercise, and smoking as well as socioeconomic factors are well-known factors of risk related to the development of CKD. Systemic and metabolic disorders, including hypertension, diabetes mellitus (DM), cardiovascular diseases, and gout can cause the increase of CKD and aggravation of the decline of GFR. Atrial fibrillation has recently been demonstrated to be a contributor to a quick decline in GFR as well as the CHA2DS2-VASc score (a stroke-risk stratification representation for patients who have atrial fibulation) has the ability to infer renal progression [12-15].
Many factors can lead to increased risk of CKD including: race, education, income and access to food. Pacific Islanders and African Americans typically have increased rates of diabetes, hypertension, and obesity that can lead to increased risk for developing ESKD. Gene encoding apolipoprotein L1 (APOL1) may be another contributing factor. Individuals with 2 APOL1 risk alleles are twice as likely to develop CKD. Sickle cell trait has been associated with increased kidney disease [16-18].
Risk Factors and Management Strategies of CKD Development and Progression
Figure 1: Outlines risk factors and management strategies of CKD development and progression [12].
Anatomy and Physiological Function
Kidneys are located in retroperitoneal space on both sides of the spine. The liver being on the right side of the abdominal cavity has led to the left kidney being located a little higher up than the right [19-21]. During the embryologic development, there are three sets of kidneys that are developed: mesonephros, metanephros, and pronephros. Beginning to form around the fourth week, the pronephros will not form into a functioning kidney. The metanephric kidney can excrete urine into the amniotic fluid in the third week of fetal development [1].
Each kidney typically weighs between 125 to 175 grams for males and 115 to 155 grams for females and measures approximately 11 to 14 centimeters in length, 6 centimeters in width and 4 centimeters thick. Approximately one third of all blood leaving the heart passes into the kidneys for filtration and is then pumped into the cells and tissues of the body [19-21]. Fat, muscles, and ribs protect the kidneys. Renal fat pat, also called perirenal fat, protects the kidneys from damage caused by external force. The renal hilum is a medial dimple that serves as the entry and exit point for nerves, ureters, vessels and lymphatics to help supply or drain the kidneys [19-21].
Anatomy of the Kidney
Blood is supplied to the kidney by the renal arteries. Specialized capillary beds called glomeruli are formed by these renal arteries that create a network of afferent arterioles that is highly specialized. Each of the glomerulus will create one part of a nephron and in order to create the efferent arterioles, capillaries will merge again. Forming a peritubular network, the efferent arterioles will twist around the tubules in the outer cortex. In the inner third of the cortex and medulla, straight long branches also known as the vasa recta will replace the peritubular network [19-20].
Figure 2: Outlines anatomy of the kidney [22].
There are 1.3 million nephrons per kidney that make up its primary functional unit. The tubules and corpuscles are the two key components of the kidney. The glomeruli are found in the corpuscles. The regulation of the passage of various chemicals into and out of the blood is done by the tubules, small tubes traveling through the inner part of the kidney. They are composed of three parts: loop of Henle, convoluted tubule, and distal convoluted tubule [1,19-20].
The outer renal tissue if found under the renal capsule and the cortex will be lighter in color. The proximal and distal convoluted tubules as well as the renal corpuscles are also found here. The cortex will reach into the inner renal tissue or medulla and will divide into renal pyramids. The loops of Henle of the renal tubes as well as the collecting ducts can be found in the renal pyramids [1-19-20].
Collecting through the minor calyx, the urine is formed inside the pyramids. Several minor calices combined together will end up forming the major calyx. The urine will move toward the renal pelvis through the major calices. The renal pelvis is a funnel-shaped structure that will be formed at the connection of all major calices [1-19-20].
Kidney Disease Staging
The disease progression helps classify kidney diseases: acute kidney injury (AKI), chronic kidney disease (CKD), and end-stage kidney disease (ESKD) [23]. Presenting a serious clinical problem, AKI can be explained as an abrupt decline in kidney function. There is a slightly larger percentage of children (34%) compared to adults (22%) across the world who will be diagnosed with AKI during hospitalization. Intensive care unit patients have the potential to have mortality rates that exceed 50%. Environmental pollutants, ischemia, and nephrotoxic medications, which have the potential to lead to apoptosis, cell damage, inflammation, vascular dysfunction, and oxidative stress can cause damage to cells within the nephron [24]. Acute kidney injury can lead to the potential recovery and regeneration of tissue that is injured or to CKD. This is known as the AKI-to-CKD transition [25]. Several patients who were able to survive the acute phase of AKI will not recover the normal function of their kidneys. This can lead to the development of ESKD and CKD. There is a complicated correlation between CKD and AKI: CKD increases the risk of AKI and AKI has the potential to lead to CKD [26-27-40].
The impact of CKD on patients is substantial as it has a 10% rate of occurrence in adults all across the world, and a rise in the rate found with the population that are aging [28]. CKD is found to exist in higher rates in countries with low and middle income. Hypertension, infection, glomerulonephritis, and environmental exposures in countries that are still developing is likely attributed to CKD. The risk of CKD has also been linked to a variety of genetic factors. Common in people with African ancestry, the 2 APOL1 risk alleles and sickle cell trait has been seen to double the risk of CKD [16]. CKD can be classified into stages 1-5 according to the National Kidney Foundation’s Kidney Disease Outcomes Quality Initiative (NKF-KDOQI). In stages 1 and 2 of CKD, the function of the kidney will experience mild changes in the glomerular filtration rate (GFR) and proteinuria. As the kidney will start to climb into stages 3 and 4, there will be about 50% of a normal kidney function. Stage 5 experiences a requirement for maintenance dialysis as the GFR is less than 15 mL/min/ 1.73 m2 [29-30].
Stages of Chronic Kidney Disease and Recommended Action Plan
Table 2: Outlines Stages of Chronic Kidney Disease and Recommended Action Plan [33].
When CKD reaches an advanced stage and the kidney begins to lose its ability to filter (GFR < 15 mL/min/1.73 m2), ESKD is known to occur. There is a high mortality risk in patients with ESKD as it accounts for 53% of deaths [31]. Kidney transplants or renal replacement therapies such as hemodialysis and peritoneal dialysis are required during this stage to increase the patient’s life expectancy [23]. An injured kidney that is classified as AKI will usually not have the ability to repair itself fully in the same capacity as other regenerative organs such as the liver or heart [8-9].
A kidney may undergo extracellular matrix deposition and fibroblast activation. This can potentially lead to nephron loss, a cycle of fibrogenesis, and induced development of CKD [8-9-32]. There is a 13 times increased risk of developing CKD sometime in their life in patients with AKI who can survive the acute phase [8-9]. The development of CKD can be caused by a kidney injury that has led to metabolic disorder and mitochondrial injury, epigenetic alterations, profibrogenic signal production, and capillary rarefaction [8-9].
Diagnostic Procedures
An important part of the management of patients with kidney disease is the assessment of renal function. Renal function tests can help assist with the identification of the presence of renal diseases, determining the progression of these diseases, and monitoring the overall response treatment [20]. Several clinical laboratory tests have been utilized in the investigation and evaluation of kidney function. The primary tests conducted for evaluating renal function are checking proteinuria and measuring the glomerular filtration rate (GFR) [20].
Equations for Estimating Glomerular Filtration Rate
Table 3: Outlines equations for estimating glomerular filtration rate [33].
The glomerular filtration rate (GFR) has been demonstrated to be the most effective indicator for looking at glomerular function. The GFR is the rate in millimeters per minute that which different substances found in the plasma can be filtered through the glomerulus. The clearance of substances from the blood is indicated through this.
The most commonly used endogenous marker for the assessment of glomerular function is creatine. An indicator of GFR is the calculated clearance of creatine involving a 24-hour urine collection. The creatine clearance can be calculated utilizing the formula: C= (U x V)/P. C is the clearance, V is the urinary flow rate, P is the plasma concentration, and U is the urinary concentration [16-34].
Screening can be vital to the early detection of kidney disease as the majority of patients with CKD are asymptomatic. Serum creatine measurement is used as a kidney profile test created by the National Kidney Foundation to help estimate GFR in urine (albumin to creatinine ratio) ACR. People with a history of diabetes/hypertension or those older than 60 years are recommended to partake in a risk-based approach to screening. Screening should also be considered in those with other risk factors such as obesity, kidney stones, autoimmune disease, reduced kidney mass, recurrent urinary tract infections, exposure to certain medications such as lithium or non-steroid anti-inflammatory drugs (NSAID), or weight loss [16,34]. Certain clinicians should inquire about more symptoms that might lead to the belief of a systemic cause (hearing loss, neuropathy, rash, hemoptysis) or obstruction in the urinary pathway (frequent/incomplete bladder emptying, urinary hesitancy, rash). Other risk factors such as prior exposure to nephrotoxins (herbal remedies, phosphate-based bowel preparations), presence of comorbidities (autoimmune disease, hypertension, chronic infections), genetic risk factors (sickle cell trait), family history of chronic kidney disease, and history of nephrolithiasis/ recurrent urinary tract infections [16].
Additional clues regarding the underlying reason for CKD can be provided through a detailed physical examination. It should also include the patient’s urine volume status. Depletion of volume could cause poor oral intake, diarrhea, and vomiting. Volume overload could be due to liver failure, nephrotic syndrome, and decompensated heart failure. A patient with enlarged kidneys or flank pain should be evaluated for polycystic kidney disease, obstructive uropathy, nephrolithiasis, and pyelonephritis. Following the examination of the skin one may find palpable purpura (vasculitis, cryobulinemia, Henoch-Schonlein purpura), extensive sclerosis (scleroderma), rash (systemic lupus erythematosus, acute interstitial nephritis), and telangiectasias (Fabry disease, scleroderma). Patients that are experiencing an advanced form of CKD may exhibit skin excoriations, asterixis, muscle wasting, altered mental status, and pericardial rub [16].
Kidney Disease Diagnosis and Staging
Figure 3: Outlines kidney disease diagnosis and staging [16].
Medical and Surgical Interventions for Kidney Disease
To discuss the possibility of kidney replacement therapy and transplant evaluation, patients with advanced kidney disease should be referred to a specialist known as a nephrologist. Clinical symptoms and GFR level are two criteria that should be considered in the decision for kidney replacement therapy. Pericarditis, pleuritis due to severe uremia, and encephalopathy are urgent symptoms that should be considered. Nausea, vomiting, pericardial rub or effusion, metallic taste, altered mental status, or volume overload including pulmonary or lower extremity edema are all symptoms indicating the initiation of dialysis [16].
The diffusion or movement of solute particles across a semipermeable membrane describes the process of dialysis. Creatinine, urea, and other metabolic waste products will diffuse down the concentration gradient from the circulation into the dialysate (sodium chloride (NaCl), sodium bicarbonate (NaHCO3), deionized water, acid concentrate). The particles size will determine the rate of diffusion across the membrane during the diffusion of dialysate. Larger solute particles will typically diffuse more slowly across the membrane. The arteriovenous shunt is formed as arteries carrying oxygenated blood from the heart are connected to a vein. This strengthens the vein by forming certain muscles around the vein [35-36].
Dialysis Process
Figure 4: Outlines kidney dialysis process [36].
Hypotension, muscle cramps, cardiovascular disease (CVD) complications, and anaphylactic reactions are all side effects of dialysis. The main reason for the diseased kidney is ongoing inflammation. It typically does not respond to medications. Chronic inflammation leads to the accumulation of metabolic wastes in the body as well as affects the normal functioning of the kidneys. The kidney may slowly regain its function as the process of dialysis can help remove certain toxins from the body. The effectiveness of the dialysis treatment can depend on the patient’s health condition and age [35-36].
Kidney Transplant
The optimal therapy for ESKD is considered to be kidney transplantation. The best outcomes have been demonstrated when the living donor kidney transplantations are performed before or shortly following dialysis. Home hemodialysis, peritoneal dialysis, in-center hemodialysis, or conservative care without dialysis are all alternative therapies for ESKD. Poor candidates for peritoneal dialysis include those with unstable housing options or patients with multiple surgeries in the abdominal area with resultant peritoneal scarring present. Immediately following the transplant, patient mortality risk is higher. However, it becomes much lower within a few months than patients on dialysis. The survivability of the patient varies depending on the patient's health and the quality of the transplant organ. Younger patients with diabetes have demonstrated the greatest transplant benefit through extending their years of life. After dialysis, patients who are 20-39 years of age live an average of 8 years vs 25 years after a transplant [16-37-40].
Patients with renal failure have seen survival and long-term improvements following a kidney transplant. The infectious disease risk and patient cardiovascular risk should be assessed before undergoing a renal transplant procedure. In order to maintain a successful kidney transplant, potential immune suppression treatment is required. Following the transplant, patients should be monitored for immunosuppressant side effects, recurrent disease, and opportunistic infections [37].
Kidney Transplant
Figure 5: Shows the anatomy of a transplanted kidney [37].
The surgeon connects the transplanted kidney to the blood vessel and balder during the kidney transplant procedure. Blood will flow through an artery and into the transplanted kidney and a vein will take the filtered blood out. The urine will then flow through the transplanted ureter and into the bladder [37].
There have been some recent improvements when it comes to renal transplants. Infection awareness and prevention increase as well as stronger immunotherapy have all been utilized. Long-term toxicity can be reduced due to the increased emphasis on induction therapy and decrease of maintenance therapy. The decrease in complications can be related to the screening for donor-specific antibodies at the time of the transplant [37-41].
Smoking is one example of multiple contraindications to a solitary kidney transplant. Quitting smoking is required by most transplant centers at least 1 year prior to the transplant. There is a doubled rate of malignancy as well as a doubled risk of a cardiac event for long-standing smokers. Active human immunodeficiency virus infection, other active infections, acute malignancy, poor financial or psychosocial situation threatening adequate care following a transplant, chronic illness with short life expectancy, acute substance abuse, lung or liver disease, active coronary artery disease, advanced age, morbid obesity, habitual noncompliance, active peptic ulcer or inflammatory bowel disease, etc. are all additional contraindications for kidney transplant [37-38-41].
There has been an increase in the number of patient candidates on the kidney waiting list over the past few decades. However, the number of transplants that are performed each year has remained consistent. Only 19,000 of the 100,000 of the patients on the list were able to receive a transplant. Three to five years is the typical wait time for a kidney transplant and rose to 6 years for decreased-donor kidney organs [37-41].
The glomerular filtration rate (GFR) is estimated to be 20mL/min/1.73 m2 or less for a patient to be placed on the waiting list for a deceased-donor kidney transplant, although referral to the list can be made earlier. Typically, 10% of transplant recipients had no dialysis before transplant. This is known as a preemptive transplant. These are the patients who will typically show the most promising outcomes and are usually living donor organ recipients. When the GFR is less than 15 mL/min/1.73m is when most patients will receive a kidney transplant [37].
Discussion
Stem cells have been tested in preclinical models and clinical trials as well as shown potential in the treatment of different diseases. The isolation of human mesenchymal stem cells (MSC) has collected from various tissues: adipose tissue, umbilical cord, and bone marrow [7, 42-47]. MSCs have the qualities of multipotent cells with self-renewal, proliferative potential, and multi-lineage differentiation [48-54]. Forming a network throughout the microvasculature, MSCs are originally from renal pericytes. The regulation of immunity, promotion of damaged tissue recovery, enhancement of potential expansion, and differentiation of host cells can all be regulated through the different cytokines and growth factors that MSCs can secrete [46,48-50,55]. MSCs also possess several intrinsic properties: anti-inflammation, immunomodulation, and anti-oxidation. These are beneficial for the limitation of acute injury and for reversing chronic depression [8,9,56,57]. The modulation of renal blood flow, endothelial survivability, immunological responses, and capillary permeability are affected by MSCs [52-54,58,59]. The recovery of sites that were damaged as well as the treatment for a variety of pathological conditions (renal injury and renal failure) are helped through the use of cells from MSCs. These characteristics support MSCs as the most promising therapeutic strategy for regenerative kidney therapy [7,51,55,60].
There are existing therapies for kidney disease that will target a variety of pathogenic mechanisms. However, these therapies do not support a cure, but they rather slow down the progression of the disease. MSCs have the benefit of presenting many regenerative effects for the protection of the kidney organ while pharmacologic interventions will only target a single aspect of AKI-CKD transition process [8-9,61,62].
In preclinical studies, MSCs have demonstrated beneficial effects. In addition, extracellular vesicles (EVs) that were released by MSCs therapy became an option in various preclinical models of kidney disease due to the immune modulatory, regenerative, and anti-inflammatory properties that were potent-cell free [23,58-59,61,63,64].
Stem Cell Trials in Animals for Kidney Disease
[65] evaluated the possible role of exosome derived from MSC for their effect on diabetic nephropathy (DN) and the enhancement of autophagy activity. There were five different groups of rats that were used in this study: DN treated with exosomes; DN treated with 3-methyladenine and chloroquine (autophagy inhibitors); DN treated with 3-methlyaldeine (3-MA), chloroquine and exosome groups; DN; and the control. The renal morphology, function, and fibrosis were all evaluated. Histological restoration of renal tissues and exosomes improved renal function were seen in this study. This research demonstrated that the attenuation of DN in a rat model of streptozotocin-induced diabetes mellitus could be linked to autophagy induction by exosomes [65].
[66] investigated a canine model of acute kidney injury (AKI) to examine the improvement of renal function as well as the utilization of mesenchymal stem cells originating from the blood of the umbilical cord. The canine AKI model was developed in 14 male beagle dogs through the utilization of an IV injection of cisplatin and gentamycin. After AKI induction, the cUCB-MSCs were administered into the renal corticomedullary junction. There was then an analysis of the clinical signs, survival time, histological parameters, and blood. In this study decreased blood creatinine and nitrogen level was shown in the group treated with AKI plus cUCB-MSCS demonstrated improved histological manifestations and an extended life-span. Overall, this study suggests that an alternative therapeutic agent for canine AKI could be cUCB-MSCs [66].
[67] tested whether extracellular vesicles can attenuate renal inflammation through the utilization of the porcine model of metabolic syndrome and renal artery stenosis as well as if their cargo of anti-inflammatory cytokine interleukin (IL) could mediate this capacity. Following 16 weeks of untreated renal artery stenosis or treated four weeks earlier with one single intrarenal delivery of extracellular vesicle gathered from tissue derived from adipose autologous MSCs, ten pigs were studied. Five more pigs displaying renal artery stenosis and metabolic syndrome each received extracellular vesicles containing pre-silenced IL10 (IL10 knock-down). They decided to study glomerular filtration rate, oxygenation, and single-kidney renal blood flow in vivo and looked at renal injury pathways ex vivo. Improvement in medullary oxygenation and fibrosis as well as the attenuation of renal inflammation were due to the extracellular vesicle delivery. Compared to metabolic syndrome, glomerular filtration rate and renal blood flow fell in metabolic syndrome and renal artery stenosis. In pigs that were treated with IL10- depleted extracellular vesicles, these renoprotective effects were blunted. This study revealed that patients with renal artery stenosis and metabolic syndrome might find extracellular vesicle-based regenerative strategies to be useful [67-69].
[70] 2017 studied 2K1C rats for the therapeutic possibility of renal subcapsular mesenchymal stem cells (MSC). The functional and morphological changes of the kidney were analyzed: renin angiotensin-converting enzyme (ACE), expression of angiotensin-II type 1 (AT1R) and type 2 (AT2R) receptors, Na+ & K+ ATPase expression and activity. There were 3 effects observed. MSC therapy reduced systolic blood pressure, ACE, renin, and AT1R; it had and upregulation in podocin and AT2R; it helped restore renal Na+ & K+ ATPase expression and activity. In addition, it also decreased proteinuria, restored plasma levels in proteins, enhanced renal morphology, and decreased fibrosis and TGF-β expression in the clipped kidney. This study showed that MSC provides a good candidate for cell therapy as well as an efficient route is transplantation into a renal subcapsule [70].
[71] 2016 investigated whether at an early age diabetic nephropathy in vivo and in vitro could be prevented by exosomes retrieved from urine stem cells. There was an isolation of exosomes coming from a conditioned medium of urine-derived stem cells (USCs-Exo). In order to look at the protective effect of USCs-Exo on podocyte apoptosis, podocytes treated with USCs-Exo in vitro were cultured in high-glucose medium. This study revealed that USCs-Exo can possibly prevent podocyte apoptosis and encourage vascular regeneration and cell survival preventing injury to the kidneys from diabetes [71].
[72] When associated with injured kidneys, researched the effect of pro-angiogenesis as well as tested the factors relating to angiogenesis that would further shine light on the most likely mechanisms. There was the isolation of EVs from the medium of the human MSCs derived from the human umbilical cord. These huMSCs were later inserted intravenously into rats after unilateral kidney ischemia. The results showed that the EVs could decrease cell apoptosis while enhancing the proliferation 24 hours following one’s kidney injury. The renal function was enhanced and there was a mitigation of the histological lesion. This study demonstrated that human MSC-EVs had the ability to protect one against reperfusion/ischemic injury through pro-angiogenesis effects in HIF-1 1α independent manner. Both ribonucleic acid (RNA) and the delivery of pro-angiogenesis-related vascular endothelial growth factor (VEGF) were involved in this process [72-73].
[74] looked at the administration effects of mesenchymal stem cells (MSCs) on diabetic nephropathy (DN) progression in severe diabetes mellitus relating to animal mode. Following diabetes induction, one group received a single dose of MSCs (DM + MSCs) while the other group received the vehicle (DM). Compared to the untreated mice, there was substantial improvement in the DM + MSCs mice group in functional parameters of the kidney. There was a decrease in renal apoptotic index and the restoration of anti-inflammatory cytokine levels/progenerative factors and an increased renal proliferation index in the DM + MSC mice. This study that was conducted showed that the administration of MSC will trigger a proregenerative microenvironment within the DN kidney. This will allow for the preservation of kidney function even if the diabetes was not corrected [74-75].
[76] investigated the repair mechanisms and biological effects of MVs. Microvesicles were gathered from MSC supernatants. Treated with a transforming growth factor known as TGF-β1, the proximal tubular epithelial cells (HK-2) were obtained in vitro. In vitro the mice were separated into four different groups. The first group was the unilateral obstruction (UUO). The second and third groups were the UUO+ MSC and UUO+MV group. The final group was the control. MVs and MSCs were then injected after surgery. The results demonstrated MV was able to help reverse the morphological changes induced by the transforming growth factor- β1 (TGF-β1). It also reduced the secretion of HK2 cells and α-SMA as well as firmed the expression of E-cadherin. This study showed that Micro-vesicles (MVs) will protect the kidneys both in vitro as well as in vivo. It also demonstrated that MVs are superior to MSCs in certain areas suggesting that MVs can be a possible treatment in the cure of kidney-related diseases [76].
[77] utilized rats to research mesenchymal stem cells (MSC) and its overall effects on diabetic nephropathy as well as the potential paracrine signals that could mediate their overall action. The rats were divided into three different groups. The first two groups were the DN rats and DN rats receiving MSCs. The final group consisted of the controls. A dose of MSCs was given to 106 cells by intravenous injection. The results demonstrated that MSC therapy could substantially enhance 24-hour urinary albumin, increase angiogenic growth factor VEGF, creatinine and serum urea concentrations, and anti-apoptotic protein bc12 while reducing pro-inflammatory TNF- α, pro-apoptotic Bax, and fibro genic growth factor TGF β. The histopathology examination demonstrated the degeneration in renal tubules as well as patchy areas of minimal necrosis [77]. This study demonstrated MSCs have the ability to enhance the function of the kidney as well as the regeneration of its tissues in DN rats. This is most probable through their paracrine action via varying growth factors: TNFα,VEGF, & TGFβ. This can also be accomplished through antiapoptotic action via Bax and bc12 genes [77].
[73] examined the effect of MVs administered following ischemic AKI on kidney injury in both the chronic and acute stages. Following the isolation of MVs from a conditioned medium inserted intravenously in rats, the ischemia of the left kidney was examined for 60 minutes. The results demonstrated that there was a mitigation of renal cell apoptosis as well as enhanced proliferation following the administration of hWJMSC-MVs in acute kidney injury (AKI) rats. Following this administration inflammation was also seen to be alleviated in the first 48 hours. This study revealed that immediately following ischemic AKI, the administration of MVs could ameliorate injury to the kidney in both the chronic and acute stages. Through the suppression of CX3CL1, the anti-inflammatory property of MVs could be the mechanism. This finding establishes a significant framework for future treatment and research [72-73].
Kidney Stem Cells in Animals Research
Research Team |
Results |
Ebrahim et al (2018) |
Exosomes improved renal function and demonstrated histological restoration of renal tissues. Autophagy induction by exosomes could attenuate DN in a rat model of streptozotocin-induced diabetes mellitus |
Lee et al (2017) |
AKI plus cUCB-MSCs had decreased blood urea nitrogen and creatinine levels, showed extended life-span and improved histological manifestations. MSCs were detected around the tubules of these kidneys at the histological level. cUCB-MSCs could be an alternative therapeutic agent for canine AKI |
Eirinet al (2017) |
Extracellular vesicle delivery attenuated renal inflammation and improved medullary oxygenation and fibrosis. Extracellular vesicle-based regenerative strategies might be useful for patients with metabolic syndrome and renal artery stenosis |
Lira et al (2017) |
MSC improved renal morphology, reduced fibrosis and TGF-β expression in the clipped kidney, decreased proteinuria and restored protein plasma levels. Transplantation into a renal subcapsule is an efficient route and MSC is a good candidate for cell therapy |
Jiang et al (2016) |
In vitro, podocytes cultured in high-glucose medium were treated with USCs-Exo to test the protective effect of USCs-Exo on podocytic apoptosis. USCs-Exo may have the potential to prevent kidney injury from diabetes by inhibiting podocyte apoptosis and promoting vascular regeneration and cell survival |
Zou et al (2016) |
EVs could reduce cell apoptosis and enhances proliferation 24 hours after kidney injury. Renal function was improved and the histological lesion was mitigated. Human MSC-EVs could protect against ischemic/reperfusion injury induced kidney injury through pro-angiogenesis effects in HIF-1α independent manner, and both the delivery of pro-angiogenesis related VEGF and RNAs were involved in this process |
Ezqueret al (2015) |
DM + MSC mice presented increased renal proliferation index, decreased renal apoptotic index and the restoration of proregenerative factors, and anti-inflammatory cytokines levels. MSC administration triggers a proregenerative microenvironment in DN kidney, which allows the preservation of the renal function even if diabetes was uncorrected |
He et al (2015) |
In vitro, MV reversed transforming growth factor-β1 (TGF-β1)-induced morphological changes, and firmed the expression of E-cadherin and reduced the secretion of α-SMA in HK2 cells. Micro-vesicles (MVs) protect kidneys both in vivo and vitro, and MVs are superior to MSCs in some respects and further suggested that MVs can be a potential therapy in treatment of kidney diseases |
Abdel Aziz et al (2014) |
MSC therapy significantly improved 24 hour urinary albumin, serum urea and creatinine concentrations, increased angiogenic growth factor VEGF, and anti-apoptotic protein bcl2 while decreased the pro-inflammatory TNF-α, fibrogenic growth factor TGF β, and pro-apoptotic protein Bax. MSCs are capable of improving the kidney function and regenerating kidney tissues in DN rats most probably through their paracrine action via different growth factors such as VEGF, TGFβ & TNFα and antiapoptotic action via bcl2 &Bax genes |
Zou et al (2014) |
After administration of hWJMSC-MVs in acute kidney injury (AKI) rats, renal cell apoptosis was mitigated and proliferation was enhanced, inflammation was alleviated in the first 48 hours. Single administration of MVs immediately after ischemic AKI could ameliorate renal injury in both the acute and chronic stage, and the anti-inflammatory property of MVs through suppression of CX3CL1 may be a potential mechanism. |
Table 4: Outlines kidney stem cell research in animals [65-77].
Stem Cell Trials in Humans for Kidney Disease
[78] is currently involved with active recruitment for a research study investigating whether MSCs can improve mortality rates of patients as the recovery of the kidney in patients that have been diagnosed with severe AKI. One hundred patients that have been diagnosed with severe AKI will participate in this randomized, placebo-controlled, patient-blinded clinical trial. Participants will be assigned to receive two doses randomly of placebo (saline) or MSCs on days zero and seven. Using the Enzyme Linked Immunoassay (ELISA) kits, urinary biomarkers of kidney repair and injury will be measured. The primary result measures are the variations in the function of the kidneys within the first twenty days after the MSC infusion. Trial registration number: NCT04194671 [78].
[79] examined the efficacy and safety of MSC derived from cord-blood (CB-MSCs) in children who had multi-drug resistant idiopathic nephrotic syndrome (MDR-INS). Pediatric patients with MDR-INS were enrolled as long as they were resistant to at least two lines of therapy. On days 0, 14, and 21 allogenic CB-MSC were administered intravenously. The patients were then followed for at least a year. The primary results were related to toxicity and safety. At a year, the secondary outcome was remission. It was evaluated by the ratio of urinary protein to urinary creatine (uPr/uCr). Eleven pediatric patients with MDR-INS resistant median of 3 prior lines of therapy were enrolled. This consisted of 10 females with a median age of 13 years. There were no patients who experienced any toxicity or infusion-related adverse events. The efficacy was testable for 9 of the patients. The median u/Pr/uCr did not change substantially from the baseline at the 12-month follow-up. However, 3 patients were in partial or complete remission. This study demonstrated that CB-MSCs are considered safe in pediatric patients with MDR-INS as well as may play a role in immunosuppressive therapy. This experience can help further phase II studies addressing the efficacy of MSC in immune-mediated kidney diseases [79].
[80] investigated the efficacy and safety of autologous MSCs. They also investigated the standard therapy in KTR containing chronic active antibody-mediated rejection (AMR) proven through biopsy. After completion of standard therapy, patients containing biopsy-proven chronic active AMR were given treatment with autologous MSCs from the bone marrow. They were then followed for up to a year. The main focus was the safety through assessment of adverse events. There were additional areas of investigation. These included: the assessment of histological and immunological changes in close relation to AMR activity, assessment of the functionality of the kidney graft, and chronicity of the immunological changes assessed by conventional microscopy and molecular transcripts. There were 3 patients who had participated in this study prior to it being shut down due to adverse events. This study concluded following treatment with MSCs, AMR did not have the ability to improve. In addition, when autologous MSCs therapy was administered in the late phase following kidney transplantation, substantial adverse events were observed in one case. This requires further investigation [80].
[81] investigated patient safety related to the clinical trials of biological agents in kidney transplant recipients (KTRs) relating to chronic active antibody-mediated rejection (CAMR). This group demonstrated a clinical trial of KTRs with CAMR that did not respond to intravenous immunoglobin and rituximab undergoing a mesenchymal stem cell (MSC) treatment. To confirm the safety of patients, this study was a phase 1 clinical trial. There were two patients with CAMR that did not respond to intravenous immunoglobulin and rituximab were included. The patients were each given allogenic MSCs for four cycles through the peripheral vein in the distal part of the arm. Renal function and adverse events were observed for half a year following the final MSC infusion. There were no substantial adverse events throughout the study. Kidney/renal function was discovered to be stable during MSC treatment. However, it gradually was reduced between the last MSC infusion and the last point of the study. For half a year, no serious adverse events after allogenic MSC treatment in KTRs with CAMR refractory to intravenous immunoglobin and rituximab were found in this study. However, further studies will be needed to explain the efficacy of MSC treatment in CAMR [81].
[82] evaluated the effect of infusion of mesenchymal stem cells for Atherosclerotic Renovascular Disease (ARVD). There were 39 patients that were studied on two separate occasions separated by 3 months who had ARVD. In 21 different patients, autologous mesenchymal stem cells derived from the adipose were infused in the renal artery at 3 variable dose levels. Seven patients each received a certain dose level. Before and three months following stem cells delivery glomerular filtration rate (GFR), renal blood flow, blood pressure, tissue oxygenation, and the levels of renal vein cytokine were monitored. There was a rise following stem cell infusion for the entire stem cell treated group, the average renal blood flow in the stenotic kidney. There was a decrease in renal vein inflammatory cytokines, hypoxia, and angiogenic biomarkers following the infusion of stem cells. There was an increase in both the average two-kidney GFR and the average systolic blood pressure. Variations in blood pressure and GFR were at most in the stem cell-treated individuals who were given a high dose. There were no apparent changes in the group that only received medical treatment. This study shows the possibility for autologous mesenchymal stem cells to increase GFR and blood flow as well as attenuate injury in the post-stenotic kidneys. Some effects are demonstration to be dose-dependent as well as related to the in-vitro qualities of mesenchymal stem cells. This may direct efforts to maximize therapeutic efficacy [82].
[42] evaluated the efficacy and safety of one injection of autologous adipose tissue-derived MSC (AT-MSCs). These stem cells were studied for the treatment of CKD. At a dosage of 1 million cells/kg, these cells were infused into the veins of six patients with CKD. The patients were followed for one year before the MSC infusion as well as a single year after post-infusion. There were no patients with adverse effects to be found in the results. In patients with AT-MSCs transplants, there was a statistical enhancement in urinary protein excretion. Following the infusion of AT-MSCs the glomerular filtration rate was not significantly reduced. Findings from this pilot study indicated that infusion of autologous AT-MSCs into the vein of patients with CKD was not related to adverse effects. It also could not help patients already involved with standard medical treatment [43].
[83] evaluated the effect of pioglitazone as a way to measure in human subjects against exposure to uremic toxin P-cresol (PC) in MSCs. MSCs apoptosis was induced under conditions relating to PC exposure. The decrease of mitophagy, PC-induced dysfunction of mitochondria through augmentation of mitofusin, and mitochondrial complexes IV and I inactivation were all related to the exposure of PC. There was a significant inhibition of PC-induced apoptosis caused by the pioglitazone-treated MSCs. Pioglitazone increased mitophagy against PC exposure as well as prevented PC-induced mitofusion through the up-regulation of tensin homolog (PTEN)-induced putative kinase 1 (PINK-1) as well as phosphatase. Through the increase of cytochrome c oxidase subunit 4 (COX4) and activation of complexes I and IV, Pioglitazone could protect against mitochondrial dysfunction that was induced. This resulted in enhanced proliferation. Pioglitazone-mediated upregulation of PINk-1 was regulated by the activation of a nuclear factor κ-light-chain-enhancer of activated B cells (NF-κB). These results indicated that the protection of MSCs from PC-induced accumulated mitochondrial dysfunction is due to pioglitazone. This is done through the P-cresol exposure conditions of NF-κB–PINK-1 axis. These results indicate that MSCs treated with pioglitazone could be a possible choice for therapy using mesenchymal stem cells in patients with CKD [83].
[84] evaluated if bone marrow mesenchymal stromal cells (BMMSCs) could enhance the function of the kidney through anti-fibrotic, anti-apoptotic, and anti-inflammatory results. This study analyzed ADPKD patients’ tolerability and safety in relation to the infusion of BMMSC. There was a year follow-up after a single-arm phase I clinical trial. There were six ADPKD patients who were eligible. According to an infusion protocol, patients were given autologous cultured BMMSCs through the cubital vein. Throughout the follow-up visits the functionality of the kidney and safety issues were being examined and compared the discoveries to the baseline and one year before the intervention. Findings revealed no serious adverse events (SAE) and adverse events related to the cell (AE) were found one-year post follow-up. The was a reduction in the mean eGFR at the one-year follow-up. This trial helped demonstrate the tolerability and safety of autologous BMMSCs intravenously. The efficacy of BMMSC in patients with ADPKD should continue to be investigated in a placebo-controlled trial with a random and large sample size. [84].
[85] evaluated the effects of stem/progenitor cells relating to bioproducts. These included extracellular vesicles whose purpose was to reprogram resident cells in chronic and acute kidney disease. There were forty patients that were divided into two groups that all were in either CKD stage III or IV. There were twenty patients in the treatment group (group A) and twenty patients in the placebo group (B). Patients in group “A” received two doses of extracellular vesicles derived from MSCs. The results demonstrated that these patients showed substantial improvement in serum creatine level, blood urea and UACR, and their eGFR. These patients also demonstrated an increase in IL-10 as well as plasma levels of TGF- β1. However, the results showed they showed a decline in plasma levels of TNF-α. Participants in group B (control) demonstrated no significant effect in any of the previously listed parameters throughout the entire study. This study demonstrated that the administration of extracellular vesicle that were derived from cell-free cord-free mesenchymal stem cells were safe. This study also shows that these vesicles can also enhance kidney function and ameliorate the inflammatory immune reaction in patients with grade III-IV CKD [85].
[86] studied the efficacy and safety of allogeneic human mesenchymal stem cells (MSCs) in decreasing the recovery time of AKI postcardiac surgery. The research group consisted of a phase 2 double-blind trial with randomly selected individuals. This placebo-controlled study took place in 27 different centers across North America. This study was meant to determine the efficacy and safety of human mesenchymal stem cells (MSCs). They looked at the reduction time when it came to the recovery from AKI post-cardiac surgery. There were 156 adult patients who were randomly selected who were undergoing cardiac surgery that displayed early signs of AKI. They either received intra-aortic MSCs or a placebo. The primary means of measurement for the kidney function recovery time was the return level of postintervention creatine to its overall baseline. Between groups in 30-day all-cause mortality, there was no substantial difference that could be detected. During the follow-up, the results showed that 12 patients who had been given AC607 and 6 patients who had been given the placebo had died. There was no difference in the rated of other adverse events between the groups. The administration of allogenic MSC did not reduce the recovery time of kidney function in patients with AKI following cardiac surgery. These results contrast with the preclinical studies and provide significant information regarding the possible effects of MSCs in this setting [86].
Kidney Stem Cells in Humans Research
Research Team |
Results |
Yang et al (2022) |
Active recruitment research study investigating whether MSCs can improve renal recovery and mortality in patients with severe AKI. One hundred subjects with severe AKI will participate in this clinical trial. Participants will be randomly assigned to receive two doses of MSCs or placebo (saline) on days 0 and 7. The main outcome measures are changes in renal function levels within the first 28 days following MSC infusion.Trial registration number: NCT04194671 |
Morello et al(2022) |
No patient experienced any infusion-related adverse event or toxicity. At the 12 months follow-up after the treatment, the median uPr/uCr did not change significantly from baseline, while 3 patients were in partial or complete remission. CB-MSCs are safe and may have a role in the immunosuppressive therapy of pediatric patients with MDR-INS. |
Veceric-Haler et al (2022) |
Areas of investigation included: safety by adverse events, assessment of kidney graft function, immunological and histological changes related to AMR activity and chronicity assessed by conventional microscopy and molecular transcripts. Three patients were enrolled in the study before it was terminated prematurely because of adverse events. AMR did not improve in any of the patients after treatment with MSCs. Serious adverse events were observed in one case when autologous MSCs therapy was administered in the late phase after kidney transplantation, which requires further investigation. |
Ban et al (2021) |
Adverse events and renal function were observed for 6 months after the final MSC infusion. No serious adverse events during the study period. Renal function was stable during MSC treatment but gradually decreased between the final MSC infusion and the study endpoint. No serious adverse events for six months after allogeneic MSC treatment in KTRs with CAMR refractory to rituximab and intravenous immunoglobulin. |
Abumoawadet al (2020) |
For stem cell treated-cohort, mean renal blood flow in the treated stenotic kidney increased after stem cell infusion. Hypoxia, renal vein inflammatory cytokines, and angiogenic biomarkers significantly decreased following stem cell infusion. Mean systolic blood pressure significantly fell and the mean two-kidney GFR increased. Changes not observed in cohort receiving medical treatment alone. Autologous mesenchymal stem cells increase blood flow, GFR and attenuate inflammatory injury in post-stenotic kidneys. |
Villanueva et al (2019) |
No patients presented with adverse effects. Improvement in urinary protein excretion observed in AT-MSCs transplanted patients. Glomerular filtration rate not significantly decreased post-infusion of AT-MSCs. Intravenous infusion of autologous expanded AT-MSCs into CKD patients was not associated with adverse effects and could benefit patients already undergoing standard medical treatment. |
Yoon et al (2018) |
Treatment of MSCs with pioglitazone significantly inhibited PC-induced apoptosis. Pioglitazone prevented PC-induced mitofusion and increased mitophagy against PC exposure through up-regulation of phosphatase and tensin homolog (PTEN)-induced putative kinase 1 (PINK-1). Pioglitazone protects MSCs against PC-induced accumulated mitochondrial dysfunction via the NF-κB–PINK-1 axis under P-cresol exposure conditions. Pioglitazone-treated MSCs could be a candidate for MSC-based therapy in patients with CKD. |
Makhloughetal(2017) |
No cell-related adverse events and serious adverse events after 12 months of follow-up. Mean eGFR value declined at the 12-month follow-up visit. Demonstrated safety and tolerability of an intravenous transplantation of autologous BMMSCs. |
Nassar et al (2017) |
Experimental group exhibited improvement of eGFR, serum creatinine level, blood urea and UACR, increase in plasma levels of TGF-β1, and IL-10 and decrease in plasma levels of TNF-α. Control group B did not show improvement in any parameters. Administration of cell-free cord-blood mesenchymal stem cells derived extracellular vesicles is safe and can ameliorate the inflammatory immune reaction and improve the overall kidney function in grade III-IV CKD patients. |
Swaminathan et al (2017) |
A significant difference between groups in 30-day all-cause mortality was not detected. At follow-up, 12 patients who received AC607 and six patients who received placebo had died. Rates of other adverse events did not differ between groups. In these patients with AKI after cardiac surgery, administration of allogeneic MSCs did not decrease the time to recovery of kidney function. These results contrast with those in preclinical studies and provide important information regarding the potential effects of MSCs in this setting. |
Table 5: Outlines kidney stem cell research in human subjects [78-86].
Conclusion
Current treatment options for kidney disease include dialysis, organ transplant as well as the use of MSC transplantation in human and animal subjects. Dialysis and organ transplant options both carry risks that may lead to considerable medical complications. Researchers found that MSC transplantation has shown improved renal function, restoration of renal tissues, reduced fibrosis, increased renal blood flow, reduced inflammatory injury, and improved glomerular filtration rate. Additional research trials are required to further investigate the efficacy and function effects for transplantation of stem cells for human patients with kidney disease including: increased population size of patients with kidney disease, information regarding specific procedures including the timing for MSC transplantation, and increased data related to the long-term outcomes of kidney disease and its treatments.
References
-
https://pubmed.ncbi.nlm.nih.gov/30855923/
-
https://www.ncbi.nlm.nih.gov/books/NBK538339/
-
Hill NR, Fatoba ST, Oke JL, Hirst JA, O'Callaghan CA, et al. (2016) Global Prevalence of Chronic Kidney Disease - A Systematic Review and Meta-Analysis. PloS one, 11(7):e0158765.
-
Thi Do D, Phan NN, Wang CY, Sun Z, Lin YC. (2016) Novel regulations of mef2-a, mef2-d, and cacna1s in the functional incompetence of adipose-derived mesenchymal stem cells by induced indoxyl sulfate in chronic kidney disease. Cytotechnology. 68(6):2589-04.
-
Fraser SDS, Roderick PJ. (2019) Kidney disease in the Global Burden of Disease Study. Nat Rev Nephrology. 15(4):193–94.
-
Susantitaphong P, Cruz DN, Cerda J, Abulfaraj M, Alqahtani F, et al. (2013) World incidence of AKI: a meta-analysis. Clin J Am Soc Nephrol. 8(9):1482–93.
-
Aghajani Nargesi A, Lerman LO, Eirin A. (2017) Mesenchymal stem cell-derived extracellular vesicles for kidney repair: current status and looming challenges. Stem cell Res Ther. 8(1):273.
-
Zhao L, Han F, Wang J, Chen J. (2019) Current understanding of the administration of mesenchymal stem cells in acute kidney injury to chronic kidney disease transition: a review with a focus on preclinical models. Stem cell Res Ther. 10(1):385.
-
Zhao Y, Zhu X, Zhang L, Ferguson CM, Song T, et al. (2020) Mesenchymal Stem/Stromal Cells and their Extracellular Vesicle Progeny Decrease Injury in Poststenotic Swine Kidney Through Different Mechanisms. Stem Cells Dev, 29(18): 1190–1200.
-
Ceccotti E, Saccu G, Herrera Sanchez MB, Bruno S. (2023) Naïve or Engineered Extracellular Vesicles from Different Cell Sources: Therapeutic Tools for Kidney Diseases. Pharmaceutics. 15(6):1715.
-
Yun CW, Lee SH. (2019) Potential and Therapeutic Efficacy of Cell-based Therapy Using Mesenchymal Stem Cells for Acute/chronic Kidney Disease. IntJ Mol Sci, 20(7): 1619.
-
Yan MT, Chao CT, Lin SH. (2021) Chronic Kidney Disease: Strategies to Retard Progression. IntMolSci, 22(18):10084.
-
Bellomo R, Kellum JA, Ronco C. (2012) Acute kidney injury. Lancet. 380 :756–66.
-
McFetridge ML, Del Borgo MP, Aguilar MI, Ricardo SD. (2018) The use of hydrogels for cell-based treatment of chronic kidney disease. Clin Sci (Lond). 132(17):1977–94.
-
Chawla LS, Kimmel PL. (2012) Acute kidney injury and chronic kidney disease: an integrated clinical syndrome. Kidney Int. 82(5):516-24.
-
Beyer-Westendorf J, Kreutz R, Posch F, Ay C. (2018) The CHA2DS2-VASc score strongly correlates with glomerular filtration rate and predicts renal function decline over time in elderly patients with atrial fibrillation and chronic kidney disease. Int JCardiol. 253:71–77.
-
Chen TK, Knicely DH, Grams ME. (2019) Chronic Kidney Disease Diagnosis and Management: A Review. JAMA. 322(13):1294–04.
-
Xu H, Chen C, Hu L, Hou J. (2017). Gene-modified Mesenchymal Stem Cell-based Therapy in Renal Ischemia- Reperfusion Injury. Current Gene Ther. 17(6):453–60.
-
Glassock RJ, Rule AD. (2016) Aging and the Kidneys: Anatomy, Physiology and Consequences for Defining Chronic Kidney Disease. Nephron. 134(1):25–29.
-
https://pubmed.ncbi.nlm.nih.gov/29939598/
-
Wallace MA. (1998) Anatomy and physiology of the kidney. AORNJournal. 68(5):800–24.
-
https://www.ncbi.nlm.nih.gov/books/NBK482385/
-
Kosanović M, Milutinović B, Kutzner TJ, Mouloud Y, Bozic M. (2023) Clinical Prospect of Mesenchymal Stromal/Stem Cell-Derived Extracellular Vesicles in Kidney Disease: Challenges and the Way Forward. Pharmaceutics. 15(7):1911.
-
Guo L, Zhang T, Wang F, Chen X, Xu H, et al.(2020) Targeted inhibition of Rev-erb-α/β limits ferroptosis to ameliorate folic acid-induced acute kidney injury. Br JPharmacol. 178(2):328–45.
-
Venkatachalam MA, Griffin KA, Lan R, Geng H, Saikumar P, et al. (2010) Acute kidney injury: A springboard for progression in chronic kidney disease. Am J Physiol Renal Physiol. 298(5):F1078–94.
-
Eckardt KU, Coresh J, Devuyst O, Johnson RJ, Köttgen A,et al.(2013) Evolving importance of kidney disease: From subspecialty to global health burden. Lancet. 382(9887):158–69.
-
Eckardt KU, Bansal N, Coresh J, Evans M, Grams ME, et al. (2018) Improving the prognosis of patients with severely decreased glomerular filtration rate (CKD G4+): conclusions from a Kidney Disease: Improving Global Outcomes (KDIGO) Controversies Conference. Kidney Int. 93(6):1281–92.
-
MussoG, Cassader M, Cohney S, De Michieli F, Pinach S, et al. (2016) Fatty Liver and Chronic Kidney Disease: Novel Mechanistic Insights and Therapeutic Opportunities. Diabetes Care. 39(10):1830–45.
-
Levey AS, Coresh J, Bolton K, Culleton B, Harvey KS, et al. (2002) K/DOQI clinical practice guidelines for chronic kidney disease: Evaluation, classification, and stratification. Am J Kidney Dis. 39(2 suppl 1):S1–266.
-
Oh HJ, Nam BY, Lee MJ, Kim CH, Koo HM, et al. (2015) Decreased circulating klotho levels in patients undergoing dialysis and relationship to oxidative stress and inflammation. Perit Dial Int. 35(1):43–51.
-
Borràs M, Cambray S,Crespo-Masip M, Pérez-Fontán M, Bozic M,et al.(2018) Peritoneal Dialysis Is an Independent Factor Associated to Lower Intima Media Thickness in Dialysis Patients Free From Previous Cardiovascular Disease. Front Physiol. 9:1743.
-
Corrêa RR, Juncosa EM, Masereeuw R, Lindoso RS. (2021) Extracellular Vesicles as a Therapeutic Tool for Kidney Disease: Current Advances and Perspectives. Int J Mol Sci, 22(11):5787.
-
Gaitonde DY, Cook DL, Rivera IM. (2017 )Chronic Kidney Disease: Detection and Evaluation. Am Fam Physician, 96(12):776–83.
-
https://doctorlib.info/nephrology/kidney/26.html
-
Andreoli MCC, Totoli C. (2020) Peritoneal Dialysis. Rev Assoc Med Bras (1992). 66Suppl 1(Suppl 1):s37–s44.
-
VadakedathS, Kandi V. (2017) Dialysis: A Review of the Mechanisms Underlying Complications in the Management of Chronic Renal Failure. Cureus. 9(8):e1603.
-
Augustine J. (2018) Kidney transplant: New opportunities and challenges. Clev ClinJMed. 85(2):138–44.
-
Wu VC, Wu CH, Huang TM, Wang CY, Lai CF, et al. (2014) Long-term risk of coronary events after aki. J Am Soc Nephrol. 25(3):595–605.
-
Wu HJ, Yiu WH, Li RX, Wong DW, Leung JC, et al. (2014) Mesenchymal stem cells modulate albumin-induced renal tubular inflammation and fibrosis. PLoS ONE, 9(3): e90883.
-
Couchoud CG, Beuscart JB, Aldigier JC, Brunet PJ, Moranne OP.(2015)Development of a risk stratification algorithm to improve patient-centered care and decision making for incident elderly patients with end-stage renal disease. Kidney Int. 88(5):1178–86.
-
Lentine KL, Kasiske BL, Levey AS, Adams PL, Alberú J, et al. (2017) Summary of Kidney Disease: Improving Global Outcomes (KDIGO) Clinical Practice Guideline on the Evaluation and Care of Living Kidney Donors. Transplantation. 101(8):1783–92.
-
Villanueva S, González F, Lorca E, Tapia A, López VG, et al. (2019) Adipose tissue-derived mesenchymal stromal cells for treating chronic kidney disease: A pilot study assessing safety and clinical feasibility. Kidney Res ClinPract. 38(2):176–85.
-
Villanueva S, Ewertz E, Carrion F, Tapia A, Vergara C, et al. (2011) Mesenchymal stem cell injection ameliorates chronic renal failure in a rat model. Clin Sci. 121(11):489–99.
-
Camilleri ET, Gustafson MP, Dudakovic A, Riester SM, Garces CG, et al.(2016) Identification and validation of multiple cell surface markers of clinical-grade adipose-derived mesenchymal stromal cells as novel release criteria for good manufacturing practice-compliant production. Stem Cell Res Ther. 7(1):107.
-
Collino F, Lopes JA, Corrêa S, Abdelhay E, Takiya CM, (2019). Adipose-Derived Mesenchymal Stromal Cells Under Hypoxia: Changes in Extracellular Vesicles Secretion and Improvement of Renal Recovery after Ischemic Injury. Cell PhysiolBiochem. 52(6): 1463–83.
-
Squillaro T, Peluso G,Galderisi U. (2016) Clinical trials with mesenchymal stem cells: An update. Cell Transplant. 25(5):829–48.
-
Zhou Y, Xu H, Xu W, Wang B, Wu H, et al.(2013) Exosomes released by human umbilical cord mesenchymal stem cells protect against cisplatin-induced renal oxidative stress and apoptosis in vivo and in vitro. Stem Cell Res. Ther. 4(2):34.
-
Choi JR, Yong KW, Choi JY. (2018) Effects of mechanical loading on human mesenchymal stem cells for cartilage tissue engineering. J Cell Physiol. 233(3):1913–28.
-
Choi JR,Pingguan-Murphy B, Wan Abas WA, Yong KW, Poon CT,et al. (2015) In situ normoxia enhances survival and proliferation rate of human adipose tissue-derived stromal cells without increasing the risk of tumourigenesis. PLoS ONE. 10(1):e0115034.
-
Choi HY, Lee HG, Kim BS, Ahn SH, Jung A,et al. (2015) Mesenchymal stem cell-derived microparticles ameliorate peritubular capillary rarefaction via inhibition of endothelial-mesenchymal transition and decrease tubulointerstitial fibrosis in unilateral ureteral obstruction. Stem Cell Res Ther. 6(1):18.
-
Liao C, Chen G, Yang Q, Liu Y, Zhou T. (2022) Potential Therapeutic Effect and Mechanisms of Mesenchymal Stem Cells-Extracellular Vesicles in Renal Fibrosis. Front cellDev Biol, 10:824752.
-
Morigi M, Rota C, Remuzzi G. (2016) Mesenchymal Stem Cells in Kidney Repair. Methods Mol Biol. 1416:89–107.
-
Togel F, Hu Z, Weiss K, Isaac J, Lange C,et al. (2005) Administered mesenchymal stem cells protect against ischemic acute renal failure through differentiation-independent mechanisms. Am J Physiol Ren Physiol.289(1):F31–42.
-
Togel FE, Westenfelder C. (2012) Kidney protection and regeneration following acute injury: Progress through stem cell therapy. Am J Kidney Dis. 60(6):1012–22.
-
Birtwistle L, Chen XM, Pollock C. (2021) Mesenchymal Stem Cell-Derived Extracellular Vesicles to the Rescue of Renal Injury. IntJMolSci. 22(12):6596.
-
Lindoso RS, Collino F, Bruno S, Araujo DS, Sant’Anna JF, et al et al.(2014) Extracellular vesicles released from mesenchymal stromal cells modulate mirna in renal tubular cells and inhibit atp depletion injury. Stem Cells Dev. 23(15):1809–19.
-
Yu B, Zhang X, Li X. (2014) Exosomes derived from mesenchymal stem cells. Int J Mol Sci. 15(3):4142–57.
-
Bruno S, Chiabotto G, Camussi G. (2014) Concise review: Different mesenchymal stromal/stem cell populations reside in the adult kidney. Stem Cells TranslMed. 3(12):1451–55.
-
Bruno S, Tapparo M, Collino F, Chiabotto G, Deregibus MC, et al. (2017) Renal regenerative potential of different extracellular vesicle populations derived from bone marrow mesenchymal stromal cells. Tissue Eng Part A. 23(21-22):1262–73.
-
Jia X, Pan J, Li X, Li N, Han Y, Feng X, et al. (2016) Bone marrow mesenchymal stromal cells ameliorate angiogenesis and renal damage via promoting pi3k-akt signaling pathway activation in vivo. Cytotherapy. 18(7):838–45.
-
Lu Y, Wang L, Zhang M, Chen Z. (2022) Mesenchymal Stem Cell-Derived Small Extracellular Vesicles: A Novel Approach for Kidney Disease Treatment. IntJ Nanomedicine. 17:3603–18.
-
Lai RC, Yeo RW, Lim SK. (2015) Mesenchymal stem cell exosomes. Semin Cell Dev Biol.40:82–88.
-
Peired AJ, Sisti A, Romagnani P. (2016) Mesenchymal stem cell-based therapy for kidney disease: A review of clinical evidence. Stem Cells Int. 2016:4798639.
-
Wang Y, Guo YF, Fu GP, Guan C, Zhang X, et al. (2020) Protective effect of miRNA-containing extracellular vesicles derived from mesenchymal stromal cells of old rats on renal function in chronic kidney disease. Stem Cell Res Ther. 11(1):274.
-
Ebrahim N, Ahmed IA, Hussien NI, Dessouky AA, Farid AS, et al.(2018) Mesenchymal stem cell-derived exosomes ameliorated diabetic nephropathy by autophagy induction through the mtor signaling pathway. Cells. 7(12): 226.
-
Lee SJ, Ryu MO, Seo MS, Park SB, Ahn JO,et al. (2017) Mesenchymal stem cells contribute to improvement of renal function in a canine kidney injury model. In Vivo. 31(6):1115–24.
-
Eirin A, Zhu XY, Puranik AS, Tang H, McGurren KA,et al. (2017) Mesenchymal stem cell-derived extracellular vesicles attenuate kidney inflammation. Kidney Int. 92(1):114–24.
-
Eirin A, Zhu XY, Jonnada S, Lerman A, van Wijnen AJ, et al. (2018) Mesenchymal Stem Cell-Derived Extracellular Vesicles Improve the Renal Microvasculature in Metabolic Renovascular Disease in Swine. Cell Transplantation. 27(7):1080–95.
-
Eirin A, Lerman LO. (2021) Mesenchymal Stem/Stromal Cell-Derived Extracellular Vesicles for Chronic Kidney Disease: Are We There Yet?. Hypertension. 78(2):261–69.
-
Lira R, Oliveira M, Martins M, Silva C, Carvalho S, et al.(2017) Transplantation of bone marrow-derived mscs improves renal function and na(+)+k(+)-atpase activity in rats with renovascular hypertension. Cell Tissue Res. 369(2):287–301.
-
Jiang ZZ, Liu YM, Niu X, Yin JY, Hu B,et al. (2016) Exosomes secreted by human urine-derived stem cells could prevent kidney complications from type i diabetes in rats. Stem Cell Res Ther. 7, 24.
-
Zou X, Gu D, Xing X, Cheng Z, Gong D,et al. (2016) Human mesenchymal stromal cell-derived extracellular vesicles alleviate renal ischemic reperfusion injury and enhance angiogenesis in rats. Am J Transl Res. 8(10):4289–99.
-
Zou X, Zhang G, Cheng Z, Yin D, Du T,et al.(2014)Microvesicles derived from human wharton’s jelly mesenchymal stromal cells ameliorate renal ischemia-reperfusion injury in rats by suppressing cx3cl1. Stem Cell Res. Ther. 5(2):40.
-
Ezquer F, Giraud-Billoud M, Carpio D, Cabezas F, Conget P,et al. (2015)Proregenerative microenvironment triggered by donor mesenchymal stem cells preserves renal function and structure in mice with severe diabetes mellitus. BioMed Res. Int. 2015:164703.
-
Ezquer ME, Ezquer FE, Arango-Rodriguez ML, Conget PA.(2012)Msc transplantation: A promising therapeutic strategy to manage the onset and progression of diabetic nephropathy. Biol Res. 45(3):289–96.
-
He J, Wang Y, Lu X, Zhu B, Pei X,et al.(2015) Micro-vesicles derived from bone marrow stem cells protect the kidney both in vivo and in vitro by microrna-dependent repairing. Nephrology. 20(9):591–600.
-
Abdel Aziz MT, Wassef MA, Ahmed HH, Rashed L, Mahfouz S,et al. (2014) The role of bone marrow derived-mesenchymal stem cells in attenuation of kidney function in rats with diabetic nephropathy. DiabetolMetab Syndr. 6:34.
-
Yang Y, Gao J, Wang S, Wang W, Zhu FL, et al. (2022) Efficacy of umbilical cord mesenchymal stem cell transfusion for the treatment of severe AKI: A protocol for a randomised controlled trial. BMJ Open. 12(2):e047622.
-
Morello W,Budelli S, Bernstein DA, Montemurro T, Montelatici E, et al.(2022) First clinical application of cord blood mesenchymal stromal cells in children with multi-drug resistant nephrotic syndrome. Stem Cell Res. Ther. 13(1):420.
-
Večerić-Haler Ž, Sever M, Kojc N, Halloran PF, Boštjančič E,et al. (2022) Autologous Mesenchymal Stem Cells for Treatment of Chronic Active Antibody-Mediated Kidney Graft Rejection: Report of the Phase I/II Clinical Trial Case Series. Trans Int.35:10772.
-
Ban TH, Lee S, Kim HD, Ko EJ, Kim BM,et al. (2021) Clinical Trial of Allogeneic Mesenchymal Stem Cell Therapy for Chronic Active Antibody-Mediated Rejection in Kidney Transplant Recipients Unresponsive to Rituximab and Intravenous Immunoglobulin. Stem Cells Int.2021:6672644.
-
Abumoawad A, Saad A, Ferguson CM, Eirin A, Herrmann SM, et al. (2020) In a Phase 1a escalating clinical trial, autologous mesenchymal stem cell infusion for renovascular disease increases blood flow and the glomerular filtration rate while reducing inflammatory biomarkers and blood pressure. Kidney Int. 97(4):793–804.
-
Yoon YM, Han YS, Yun CW, Lee JH, Kim R,et al. (2018) Pioglitazone protects mesenchymal stem cells against p-cresol-induced mitochondrial dysfunction via up-regulation of pink-1. Int J Mol Sci. 19(10):2898.
-
Makhlough A, Shekarchian S,Moghadasali R, Einollahi B, Hosseini SE,et al. (2017) Safety and tolerability of autologous bone marrow mesenchymal stromal cells in adpkd patients. Stem Cell Res Ther. 8:116.
-
Nassar W, El-Ansary M, Sabry D, Mostafa MA, Fayad T,et al. (2016) Umbilical cord mesenchymal stem cells derived extracellular vesicles can safely ameliorate the progression of chronic kidney diseases. Biomater Res. 20:21.
-
Swaminathan M, Stafford-Smith M, Chertow GM, Warnock DG, Paragamian V, et al. (2018) Allogeneic mesenchymal stem cells for treatment of AKI after cardiac surgery. J Am Soc Nephrol. 29(1):260–7.