Pluripotent Stem Cells: Potential, Limitation, and Challenges
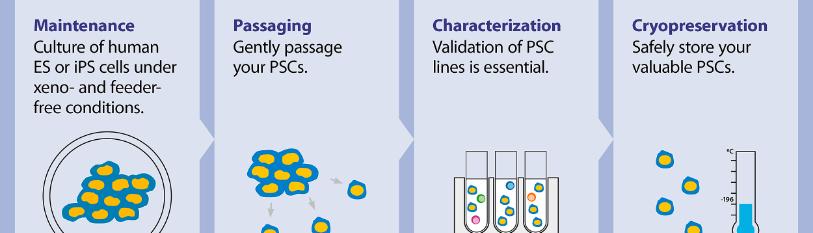
Suad Trebinjac*
1Czech Rehabilitation Hospital Al Ain, UAE- Director of Rehabilitation Department
2Al Garhoud Private Hospital, Dubai, UAE – Consultant in PM&R
3 FIFA Medical Center of Excellence, Dubai, UAE –Consultant in PM&R
*Corresponding author: Suad Trebinjac, Czech Rehabilitation Hospital Al Ain, UAE- Director of Rehabilitation Department
Citation: Trebinjac S. (2022) Pluripotent Stem Cells: Potential, Limitation, and Challenges. J Stem Cell Res. 3(3):1-7.
Received: July 11, 2022 | Published: July 29, 2022
Copyright© 2022 genesis pub by Trebinjac S. CC BY NC-ND 4.0 DEED. This is an open-access article distributed under the terms of the Creative Commons Attribution-NonCommercial-No Derivatives 4.0 International License., This allows others distribute, remix, tweak, and build upon the work, even commercially, as long as they credit the authors for the original creation.
DOI: https://doi.org/10.52793/JSCR.2021.3(3)-39
Abstract
The research of stem cells and their application in clinical practice represents one of the most exciting areas in modern science. Due to their specific properties, stem cells offer the possibility of restoring damaged tissues and the function of impaired organs. Pluripotent cells of embryonic origin as well as reprogrammed adult cells have the greatest regenerative potential, but also carry the greatest risk of tumor development and autoimmune reactions. In addition, the use of embryonic cells is ethically unacceptable because it implies the destruction of the embryo. This manuscript analyzes the potential of pluripotent stem cells, but also the limitations and challenges that scientists are currently facing
Keywords
Puripotent stem cells; Potential; Challenges
Introduction
Since Canadian scientists Ernest McCulloch and James Till, in the early 1960s, discovered hematopoietic cells able to heal blood diseases, scientific but general interest in stem cell applications has grown steadily.
Stem cells are specific cells in the human body able to divide and differentiate into other types of cells forming new tissue and restoring impaired functions. The discovery of stem cells gives hope that many currently incurable diseases will eventually be cured.
Numerous scientific papers have contributed to new knowledge about the nature of stem cells and their function, but at the same time opened many questions still waiting for an answer. The first controversy is related to the term itself. Soviet scientist Alexander Friedenstein isolated cells that under laboratory conditions present cell progenitors to fibroblasts and other mesodermal fold cells [1]. Caplan named these cells “Mesenchymal stem cells” [2], but after 25 years the same author suggested other name “Medicinal Signaling Cells” [3].
The main reason for rename is the adoption of the hypothesis of paracrine action of stem cells rather than direct transformation into the "new cells", at the site of damaged tissue.
This advanced knowledge has stimulated thinking about the real potential of stem cells, the limits of their action, and the challenges facing science.
Therapeutic potential of stem cells
Stem cells are undifferentiated cells that under certain circumstances can differentiate into all types of cells. This allows them to eventually regenerate damaged tissues and restore the body's function [4] They can be classified in different ways (Figure 1).
Embryonic, infantile, and adult cells consider therapeutically efficient. Recently, reprogrammed adult cells with similar properties to embryonic cells have attracted attention. They are named induced pluripotent stem cells (iPSCs).
Figure 1: Classification of Stem Cells.
Human Embryonic stem cells (hESCs)
Due to their pluripotent properties, cells of embryonic origin probably have the greatest therapeutic potential. However, their use is prohibited because isolation of cells requires the destruction of the embryo, and in addition, carries the risk of rejection and malignancy [5].
Embryonic stem cells are for the first time derived from an inner layer of blastocyst by Thompson’s group in 1998 [6,7]. They used in vitro fertilized eggs for obtaining cells. These pluripotent cells possess the properties of self-renewal and proliferation into all germ layers. They can differentiate into more than 200 types of cells originating from the endoderm, ectoderm, and mesoderm [8,9].
Preclinical studies offered promising results. Animal experiments have confirmed that transplantation of hESCs leads to the formation of oligodendrocyte progenitors and the restoration of motor function after spinal cord injury [10]. Successful clinical application of hESCs was reported in 2015 in the treatment of age-related macular degeneration and Stargardt’s macular dystrophy. In two prospective studies after 22 months, most patients had improved vision compared with the control group. No significant adverse reactions were reported [11].
Ethical dilemmas related to the application of embryonic stem cells remain the subject of intense discussions involving not only scientists but also lawmakers, religious authorities, and politicians. The problem is attempted to be overcome by using embryos through in vitro fertilization (IVF). However, the moral dilemma of whether embryos are future humans even produced in vitro is still the subject of debate and it is treated differently in different countries.
Induced pluripotent stem cells (iPSCs)
Considering above mentioned challenges, scientists' research was aimed at reprogramming adult cells that would have similar properties to embryonic ones, but without ethical implications or potential health risks.
In 2006, Japanese scientists Takahashi and Yamanaka succeeded in reprogramming mouse cell fibroblasts by giving them embryonic cell properties. They achieved this by adding four pluripotency-associated genes, the so-called Yamanaka factors: Oct3/4, SOX2, c-Myc, and Klf4. These cells are named induced Pluripotent Cells (iPSCs) [12]. A similar result was replicated in humans. At the same time, another group of scientists (Thomson’s group) announced the invention of human iPSCs by using different factors [13].
The main advantage of iPSCs would be in their maintenance of pluripotency without ethical consequences. They can be used for regeneration of damaged tissues, but also for the analysis of molecular changes in various diseases, as well as for the examination of toxic and other properties of pharmacological substances.
iPSCs can be obtained by reprogramming any somatic cell. The whole process can be divided into 3 phases:
-isolation and culturing of cells,
- transfected cells with transcription factors and,
- analysis of cells and their expansion (14) (Figure 2).
Figure 2: Process of Generation of iPSCs [14].
(1) Establishment of culture: the source cells are cultured for further use as host cells for the delivery of reprogramming proteins. (2) The cultured source cells are then transfected with the four factors from Yamanaka's cocktail and incubated on feeder layers that provide the nourishment to host cells and are responsible for the formation of extra cellular matrix, under suitable conditions of media. Two types of methods for the delivery of reprogramming factors into the somatic cells can be used- Integrating viral vector systems and Non-Integrating methods. (3) After the formation of iPSCs, they are characterized by different morphological and physicochemical analyses, which is followed by the expansion of iPSCs.
Transcription factors can be introduced into the somatic cell in two ways: through Integrating Viral Vector Systems and Non-Integrating Systems. The integrated system involves the use of retro and lentil viruses that are integrated into the genome of a somatic cell via a vector [15]. The non-integrated method involves the use of "non-integrated substances" such as Adenovirus and Sendai virus, Plasmid DNA, RNA, and proteins [14].
It was confirmed that mouse fibroblasts modified with OCT4, SOX2, KLF4, and MYC factors show a tendency to proliferate into glial and neural progenitors that migrate and differentiate into glia and neurons when injected into the embryonic cortex [16].
iPSCs have been used in animal studies to treat Parkinson's disease [17], platelet deficiency [18], spinal cord injury [19] macular degeneration [20], and many other conditions.
The first clinical application was conducted at the RIKEN Institute in Japan in 2014 on patients with Macular degeneration [21]. Slowing down of the disease without significant adverse or immunological reactions was recorded in the first patient. However, the study was stopped in another patient for safety reasons [22]. Since that time several clinical studies using allogeneic sources of iPSCs have been approved. The most of ongoing research are nationally approved in Japan. The studies are related to Parkinson’s disease, AMD, severe cardiac failure, aplastic anemia, spinal cord injury, and corneal stem cell deficiency [23].
The application of iPSCs faces a lot of challenges. Using the integral viral system initially produced less than 1% iPSCs. It was also discovered that the insertion of lentiviruses and retroviruses as a part of the integration process leads to mutagenesis [24]. Two Yamanaka factors, c-Myc and Klf4 have pluripotent but also oncogenic properties [25]. Another Yamanaka factor Oct3/4 also play the role in the development of different cancers including pancreatic cancer, gastric cancer, oral squamous cell carcinoma, seminoma, and bladder cancer [26-28].
The majority of these problems were solved by the implementation of “nonintegrated” factors [29,30].The preparation of iPSCs by using autologous sources, although immunologically safe, is a very slow and time-consuming process, unacceptable for widespread application [31]. The allogeneic sources are susceptible to autoimmune reactions due to the incompatibility of the donor's HLA system with recipients. The use of immunosuppressive agents is followed by adverse reactions [32]. There is an attempt to solve the problem by forming the cell banks by matching the HLA of donors with the majority of the population.
Conclusion
Interest in the application of pluripotent stem cells is constantly increasing, which is confirmed by the growing number of published studies [33].
The possibility of reprogramming adult cells opened up the prospect of regenerating damaged tissues and restoring function without ethical implications. Preclinical research and initial clinical results are encouraging, albeit many challenges that prevent more frequent clinical applications are still present.
It expects that the selection of the most appropriate cells, the development of new technologies, and a better understanding of the interactions of pluripotent cells with the host environment will contribute to the safer application and better therapeutic outcomes.
References
- Friedenstein AJ, Piatetzky S, Petrakova KV. (1966) Osteogenesis in transplants of bone marrow cells. J Embryol. Exp Morphol. 16:381-90.
- Caplan AI. (1991) Mesenchymal stem cells. J Orthop Res. 9(5):641-50.
- Caplan AI. (2017) Mesenchymal Stem Cells: Time to Change the Name! Stem Cells Translational Medicine. Stem Cells Transl Med. 6(6):1445-51.
- Biehl JK, Russell B. (2009) Introduction to Stem Cell Therapy. J Cardiovasc Nurse. 24(2):98-105.
- Zhang M, Cheng L,Yuyan J. (2016) Aneuploid embryonic stem cells exhibit impaired differentiation and increased neoplastic potential. EMBO J. 35(21):2285-300.
- Thomson JA, Itskovitz-Eldor J, Shapiro SS, Waknitz MA, Swiergiel JJ, et al. (1998) Embryonic stem cell lines derived from human blastocysts. Science. 282(5391):1145-7.
- Keller. (2005) Embryonic stem cell differentiation: emergence of a new era in biology and medicine. Genes Dev. 19:1129-55.
- Itskovitz-Eldor J, Schuldiner M, Karsenti D, Eden A, Yanuka O, et al. (2000) Differentiation of human embryonic stem cells into embryoid bodies compromising the three embryonic germ layers. Mol Med. 6(2):88-95.
- Wray J, Hartmann Ch. (2012) WNTing embryonic stem cells. Trends Cell Biol. 22(3):159-68.
- Keirstead HS, Nistor G, Bernal G. (2005) Human Embryonic Stem, Cell-Derived Oligodendrocyte Progenitor Cell Transplants Remyelinate and Restore Locomotion after Spinal Cord Injury. J Neurosci. 25(19):4694-705.
- Schwartz DS, Regillo C, Lam LB, Eliott D, Rosenfeld PJ, et al. (2015) Human embryonic stem cell-derived retinal pigment epithelium in patients with age-related macular degeneration and Stargardt's macular dystrophy: follow-up of two open-label phase 1/2 studies. Lancet. 385(9967):509-16.
- Takahashi K, Yamanaka S. (2006). Induction of pluripotent stem cells from mouse embryonic and adult fibroblast cultures by defined factors. Cell. 126:663-76.
- Yu J, Vodyanik AM, Smuga-Otto K, Bourget JA, Frane JL, et al. (2007). Induced pluripotent stem cell lines derived from human somatic cells. Science 318,1917-20.
- Vimal SK, Manish K, Neeraj K, Abhishek S, Ramesh C. (2015) Induced pluripotent stem cells: applications in regenerative medicine, disease modeling, and drug discovery. Front Cell Dev Biol. 3:2.
- Takahashi K, Ichisaka T, Yamanaka S. (2006). Identification of genes involved in tumor-like properties of embryonic stem cells. Methods Mol Biol. 329:449-58.
- Wernig M Zhao JP, Pruszak J, Hedlund E, Fu D, Soldner ,F et al. (2008) Neurons derived from reprogrammed fibroblasts functionally integrate into the fetal brain and improve symptoms of rats with Parkinson’s disease. Proc Natl Acad Sci. 105(15):5856–61.
- Kriks S, Shim JW, Piao J, Ganat YM, Wakeman DR, et al. (2011) Dopamine neuronsderived from human ES cells efficiently engraft in animal models of Parkinson’s disease. Nature 480:547-51.
- Takayama N, Nishimura S, Nakamura S, Shimizu T, Ohnishi R, et al. (2010). Transient activation of c-MYC expression is critical for efficient platelet generation from human induced pluripotent stem cells. J Exp Med. 207(13):2817-830.
- Nori S, Okada Y, Yasuda A, Tsuji O, Takahashi Y, et al. (2011). Grafted human induced, pluripotent stem-cell-derived neurospheres promote motor functional recovery after spinal cord injury in mice. Proc Natl Acad Sci USA. 108:16825-830.
- Okamoto S, Takahashi M. (2011) Induction of retinal pigment epithelial cells from monkey iPS cells. Invest Ophthalmol Vis Sci. 52(12):8785-879.
- Eguizabal C, Aran B, Chuva de Sousa Lopes SM, Geens M, Heindryckx B, Panula S, et al. (2019) Two decades of embryonic stem cells: A historical overview. Human Reprod Open. 2019(1):1-17.
- Mandai M, Watanabe A, Kurimoto Y, Hirami Y, Morinaga C, Daimon T, et al. (2017) Autologous induced stem-cell-derived retinal cells for macular degeneration. The New England J Med. 376:1038-46.
- https://www.intechopen.com/books/8026
- S Yamanaka. (2009) Elite and stochastic models for induced pluripotent stem cell generation. Nature. 460(7251):49-52.
- Klimczak M. (2015) Oncogenesis and induced pluripotency – commonalities of signalling pathways. Contemp Oncol (Pozn). 19(1A):A16-A21.
- Wasik AM, Grabarek J, Pantovic A, Pobuda AC, Asgari HR, et al. (2014) Reprogramming and carcinogenesis-parallels and distinctions. Int Rev Cell Mol Biol. 308:167-203.
- Koide H. (2014) Embryonic stem cells and oncogenes. Pluripotent Stem Cell Biology-Advances in Mechanisms, Methods and Models. InTech. 2014:41–61.
- Lu Y, Zhu H, Shan H, Lu J, Chang X, et al. (2013) Knockdown of Oct4 and Nanog expression inhibits the stemness of pancreatic cancer cells. Cancer Lett. 240:113–23.
- Fusaki N, Ban H, Nishiyama A, Saeki K, Hasegawa M. (2009) Efficient induction of transgene-free human pluripotent stem cells using a vector based on Sendai virus, an RNA virus that does not integrate into the host genome. Proc Jpn Acad Ser B Phys Biol Sci. 85(8):348-36.
- Warren L. (2010) Highly efficient reprogramming to pluripotency and directed differentiation of human cells with synthetic modified mRNA. Cell Stem Cell 7(5):618 -30.
- Bravery CA. (2015) Do human leukocyte antigen-typed cellular therapeutics based on induced pluripotent stem cells make commercial sense? Stem Cells Dev. 24(1):1-10.
- Malat G, Culkin C. (2016) The ABCs of Immunosuppression: A Primer for Primary Care Physicians. Med Clin N Am. 100:505-18.
- Kobold S, Guhr A, Mah N. (2020) A Manually Curated Database on Clinical Studies Involving Cell Products Derived from Human Pluripotent Stem Cells. Stem Cell Reports. 15(2): 546-55.