Immunomodulatory Effects of Mesenchymal Stem Cells Derived from Bone Marrow, Adipose Tissue, and Umbilical Cord Tissue in Rheumatoid Arthritis
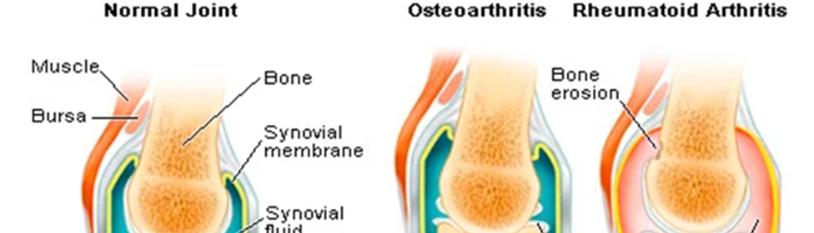
Brooke Billings and Vincent S Gallicchio*
Department of Biological Sciences, College of Science, Clemson University, Clemson, South Carolina, USA
*Corresponding author: Vincent S Gallicchio, Department of Biological Sciences, College of Science, Clemson University, Clemson, South Carolina, USA
Citation: Billings B, Gallicchio VS. (2021) Immunomodulatory Effects of Mesenchymal Stem Cells Derived from Bone Marrow, Adipose Tissue, and Umbilical Cord Tissue in Rheumatoid Arthritis. J Stem Cell Res. 2(1):1-22.
Received: December 08, 2020 | Published: December 25, 2020
Copyright© 2020 genesis pub by Bilings B, et al. CC BY-NC-ND 4.0 DEED. This is an open-access article distributed under the terms of the Creative Commons Attribution-Non Commercial-No Derivatives 4.0 International License., This allows others distribute, remix, tweak, and build upon the work, even commercially, as long as they credit the authors for the original creation.
DOI: https://doi.org/10.52793/JSCR.2020.2(1)-13
Abstract
Rheumatoid Arthritis (RA) is an autoimmune disease that targets the synovial joints of the body that holds a massive burden on healthcare worldwide. The progression of the disease is mediated in congruenceof the adaptive immune cells (T- and B- cells) and synovial fibroblast activation. In the last decade, standard treatment options for RA have demonstrated significant improvements but often fail to deliver a long-term therapeutic outcome and, in some cases, cause adverse effects leading to treatment termination. Treatment with mesenchymal stem cells (MSCs) has been recently studied in RA because of the unique immunomodulatory and anti-inflammatory capacities of the MSCs needed to combat RA. Pre-clinical and clinicaltrials have demonstrated that MSCs derived from adipose tissue, bone marrow, and umbilical cord tissue possess immunomodulatory capabilities that alter thedysfunctional immune cells involved with the pathogenesis of autoimmune diseases including RA. Specifically, thesetrials have demonstrated the immunomodulation ability of MSCs on the proliferation of memory T cells as well as their ability to migrate to inflamed tissue associated with synovitis in RA.
Keywords
Rheumatoid arthritis; Mesenchymal stem cells; Autoimmune disease; Inflammation; Synovial joint
Abbreviations
RA: Rheumatoid arthritis; OA: Osteoarthritis; MSC: Mesenchymal stem cell; hESC: Human embryonic stem cells; ICM: Inner cell mast; ADC: Adult stem cell; AD-MSC: Adipose-derived mesenchymal stem cell; UC-MSC: Umbilical cord derived mesenchymal stem cell; BM-MSC: Bone marrow derived mesenchymal stem cell; WJ: Wharton’s Jelly; MHC: Major histocompatibility complex; HLA: Human leukocyte antigen; RF: Rheumatoid factor; ACPA: Anti-citrullinated peptide antibodies; APC: Antigen-presenting cell; Ig: Immunoglobulin; DMARD: Disease-modifying anti-rheumatic medications; IGF: Insulin-like growth factor; TGF: Transforming growth factor; BMP: Bone morphogenetic proteins; SVF: Stromal vascular fraction; IL-17: Interleukin 17; Th17: T helper 17 cell; TNF: Tumor necrosis factor; NO: Nitric oxide; CIA: Collagen-induced arthritis; NSAID: Nonsteroidal anti-inflammatory drugs; MMP: Matrix metalloproteinases; Nk: Natural killer cell; TLR: Toll-like receptor; COMP: Cartilage oligomeric matrix protein; DMEM medium: Dulbecco/Vogt Modified Eagle’s Minimal Essential Medium; CFA: Complete Freund’s adjuvant
Introduction
Rheumatoid Arthritis (RA) is a form of arthritis that can cause swelling, stiffness, pain, damage, and loss of functionin the joints of the body. The complexity of the elements responsible for the onset of RA contribute to the challenges of proper prevention, diagnosis, and treatment for this disease.The commencement of the autoimmune disease is influenced bya combinationof genetic, epigenetic, and environmental risk factors. The progression ofcartilage and bone erosion are consequences of inflammation of synovial joint membranes. Unlike osteoarthritis, primarily a degenerative disorder resulting from mechanical wear of the joints, symptoms associated with RAarise from an autoimmune disorder [1]. Autoimmune disorders are diseases in which the immune system mistakenly attacks and damages its own tissues.Moreover, RA is a systemic inflammatory autoimmune disorder, meaning the autoantibodies that target the joint tissues do not discriminate and target joints on both sides of the body equally [2]. The target tissue associated with RA is found within synovial joints. These joints are unique to the human body in that they are comprised of ajoint cavity designed to permit the highest range of joint motion. The cavity is a fibrous capsule lined by an inner synovial membrane. Synovial membranesfunction in maintaining stability, permitting space between articulating bones, and reducing friction amongst the moving bones [3]. This is accomplished by thesecretion of synovial fluid into the joint cavity, which is necessary in maintaining a smooth, gliding synovial joint. Resident fibroblast-like synoviocytes housed within the membrane aid in synovial fluid production and retaining homeostatic mechanisms of the joint cavity and the synovial membrane itself.The fibroblasts in RA are altered to excessively proliferate while increasing their secretion of cytokines and proteases. Also present are permanent synovial macrophages that under normal conditions remain quiescent, unlike synovial macrophages of patients with RA. The synovial macrophageinvolvement in the pathogenesis of RA is observed bytheir influence in activating the proliferation of fibroblasts and secretion of cytokines. On the surface of the two bones is a layer of hyaline (articular) cartilage to provide additional support to the moving bones. Chondrocytes, exclusive to articular cartilage, regulate the continuous remodeling of the extracellular matrix in the cartilage. In RA, the release of pro-inflammatory cytokines by the resident cells of the synovium induce the chondrocytes to increase secretion catabolic tissue enzymes and inhibition of tissue repairing mechanisms resulting in cartilage damage. Together, these cells collectively interact and contribute to the development of RA related inflammation, cartilage deterioration, and bone erosion [4] (Figure 1) .
Figure 1: A comparative diagram displaying the anatomical features of a normal synovial joint (left), a synovial joint inflicted by osteoarthritis (middle), and a synovial joint inflamed due to rheumatoid arthritis (right). Synovial membrane inflammation is a key characteristic of RA patients unlike OA. Shown in the RA synovial joint, the synovial membrane is red, thickened, and larger in size representing the inflammation. The inflamed synovial membrane is much larger than in a normal synovial joint and OA synovial joint [4,5]
In RA, inflammation primarily occurs in the synovial membranes of smaller synovial joints such the wrists, fingers, and feet observed by cardinal signs of inflammation. Chronic inflammation of the synovial membrane progressively alters the structure and function of synovial joints. Overall, this results in destruction of the tissues of the joint cavity which exposes the articulating bones causing detrimentalbone erosion [5]. Historically, the first diagnosis of this autoimmune disease is one of the oldest that still prevails. Despite the large amount of historical evidence suggestive of RA, the first formal evidence of RA appeared in 1591.The French physician Guillaume de Baillou applied the age-old term “rheumatism” to a musculoskeletal condition characterized by swelling, stiffness, and pain [6]. The modern diagnosis and description of RA is credited to Sir Alfred Garrod in 1852. Garrod renamed the condition described by Guillaume torheumatoid arthritis and defined it as a separate condition from osteoarthritis. As it is presently known on a global perspective, 80% of RA patients encompass the ages of 35-50 years. Furthermore, studies have shown that women are 3 times more likely to be diagnosed with RA than men [7]. Although the exact mechanisms behind the course and prognosis differences of RA among males and females have yet to be revealed, gender differences in hormone production, gender-based roles, physiological characteristics, and chromosomal composition have been proposed [8] (Figure 2).
Figure 2: Display of structural comparison between the x-ray images of synovial joints in the fingers, hand, and wrist of healthy joints (left) and x-ray images of bone erosion (right) imposed on synovial joints in patients with RA [10].
Rheumatoid arthritis presents varying clinical symptoms depending on the maturity and severity of the disease. In general, clinical symptoms associated with RA are often worse in the morning due to stiffness of the inflamed joints, but pain may be elevated during the day through physical activity tohelpreduce inflammatory debris inside the joint cavity [9]. RA is broadly characterized into four stages: [a] early stage RA, [b] moderate stage RA, [c] severe stage RA, and [d] end-stage RA [10]. Early stage RA is characterized by synovitis, which occurs when the synovial membrane of synovial joints become inflamed and condensed. Common symptoms of early stage RA include swollen joints, joint stiffness, fatigue, fever, joint tenderness, and loss of appetite. When RA emerges, the immune system generates an attack on the synovium (synovial membrane) causing a release of proteins that prompt blood vessels in the synovium to multiply, referred to as angiogenesis [11]. This process aids the progression of the immune response by allowing more influx of immune cells thus permitting further inflammation and excessive proliferation of fibroblasts in the synovial membrane.Additionally, the excessive tissue growth is aided in part by more nutrients supplied by angiogenesis.In RA, the intense cellular infiltration of inflammatory/immune cells in combination of the accompanied angiogenesis, allows the formation of the hypertrophied synovium. The onset of moderate stage RA is marked by pannus formation. The hypertrophied synovium, called the pannus, is the invasive and enlarged synovial membrane due to increased proliferation of synovial fibroblast-like cells [12]. The symptoms of patients in moderate stage RA experience a worseningof joint pain, swelling, tenderness and stiffness. As RA progresses, the continuing inflammation and fibroblast proliferation of the pannus spreads across the articulating bones and may lead toheightened deterioration of the joint cavity. Severe stage RA is characterized by destruction of the fibrous capsule and bone erosion. Due to the deterioration of the cushion between the bones, the bones rub together causing damage, erosion, and visible joint deformities. Symptoms associated with severe stage RA include increased pain and swelling, reduced mobility, and muscle weakening. The last stage is end-stage or terminal RA. This is characterized by the complete deterioration of the synovial joint cavity, cartilage depletion, and bone fusion. This is most detrimental form of RA in that the damage is typically irreversible with standard treatment options and often requires surgical interventions [13].
A complete pathological portfolio of RA is yet to be determined;however,genetic, epigenetic, and environmental factors areimplicated as strong contributors. Smoking and pathogenic infectionshave been observed to induce epigenetic changes as well as direct interactions on the development, rate of progression, and severity of RA [14]. Therefore, it is appropriate to classify RA as an immune-mediated disease with a strong genetic influence that may be induced by environmental factors.The present study will postulate that RA starts with a high-risk genetic background that, in combination with environmental exposures, launches a cascade of events through various immune cell subsets and signaling pathways inducing inflammation in synovial joints and ultimately chronic destructive arthritis. The adaptive immune cells (B-cells, T-cells and macrophages) play critical roles in autoimmune disease pathogenesis [15]. In relation to RA, these cells reside either in the synovial membrane or in blood circulation. Within the last decade, notable studies have been carried out with the effortof understanding the role of T-cells in RA [16].There are two major types of T-cells, T-helper (CD4+) cells and cytotoxic T-cells (CD8+). As the name implies, CD4+ T-cells help the immune system carry out its proper functions by stimulating other immune cells and CD8+ T-cells kill virally infected cells. T-cells constitute the cell-mediated immune reactions in the human body by recognizing degraded fragments of foreign molecules (antigens) expressed by antigen-presenting cells (APCs) by a highly variable T-cell receptor expressed on the T-cell surface[17]. For T-helper and cytotoxic T-cells to perform their appropriate functions, they must be activated to proliferate and differentiate into effector cells via the interaction with antigen-presenting cells (APCs). Under normal human physiological conditions, APCs can detect and degrade foreign antigens into protein fragments that bind to MHC proteins within the cytoplasm. These MHC proteinscarry the bound foreign antigens fragments to the cell surface as a presentation for T cells [18]. MHC proteins are encoded by a large complex of genes referred to as the major histocompatibility complex which are the fundamentals in organ transplantations.
There are two classes of MHC proteins. Class I MHC proteins present foreign peptides to cytotoxic T cells and class II MHC proteins present foreign peptides to T helper cells [19]. Class I MHC proteins are generally expressed on all nucleated cells of the human body. In contrast, class II MHC proteins are primarily localized to APCs that uptake foreign antigens in extracellular fluid such as B-cells and macrophages [20]. Dendritic cells are unique because they display both class I and II MHC proteins meaning they can interact and activate both CD4+ and CD8+ T cells [21]. One of the difficulties in determining the genetic association responsible for the immune cell imbalance in RA is simply that this is a heterogenous disease resulting from a variety of pathways and risk factors. However, a consistent trend implicated in the literature suggests that one of the major genetic risk factors associated with developing RA in humans is the presence of the gene that codes for the human leukocyte antigen called HLA-DR4 (HLA-DRB1-0401) [22-24]. The two main autoantibodies that have been associated with the joint tissue attack in RA are Rheumatoid Factor (RF) and anti-citrullinated peptide antibodies (ACPAs). RF is an autoantibody that is an isotype of IgM antibodies that targets the Fc domain on altered human IgG antibodies. Anti-citrullinated peptide antibodies have been observed in a wide variety of patients with RA which target certain peptides containing citrullinated arginine residues[25]. The exact origin of these autoantibodies is unknown, but the literature implicates a strong link between the development of ACPAs and the presence ofan HLA-DR4 shared epitope and smoking [26]. The cause of the cascade of mechanisms behind the purpose of these autoantibodies are generally thought to occur by modification of IgG and citrullinated proteins found in Type-II collagen and vimentin. These modifications cause confusion of antigen presenting cells which no longer recognize these as self-antigens [27]. The APCs carry the antigens to the lymph nodes to activate CD4+ T cells which lead to stimulation of B cells. These B cells begin to proliferate and differentiate into plasma cells which produce specific autoantibodies responsible for targeting the self-antigens in RA. Following this, CD4+ T cells and antibodies travel through blood circulation and reach their targets in the synovial joint. There, T helper cells secrete cytokines such as Interferon-g and Interleukin-17 to recruit other immune cells while inducing synovial macrophages to produce more cytokines such as TNF-a, Interleukin-1, and Interleukin-6 [28]. The combined effects of these cytokines produced by T helper cells and macrophages stimulate fibroblast proliferation. Also, fibroblasts increase production of proteases which overtime, alongside the gradual growth of the pannus, leads to cartilage damage and destruction [29]. The proteases degrade the articular cartilage, thereby removing the protective covering of articulating bones and causing bones to rub against each other. Recent advancements in establishing an appropriate target for treatment in RA implicate that further destruction of synovial joints is linked to a receptor-mediated pathway between T cells present within the synovial cavity and osteoclasts leading to stimulation of bone resorption (breakdown) by osteoclasts [30]. The function of B-cells in the progression of RA is noted by their physiological secretion of autoantibodies rheumatoid factors (RF) and anti-citrullinated protein antibodies (ACPA) [31]. When in the synovial fluid, RFs target altered IgG antibodies and ACPAs target citrullinated proteins and bind to them forming immune complexes. These immune complexes activate the complement systemwhich activates inflammation and tissue damage of the joint [32].
The standard methods for diagnosing RA include blood sampling to detect levels of the auto-antibodies RF and ACPAs. X-ray examinations may be used to observe a narrowing of the joint space, soft tissue swelling, and decreased bone density [33]. The current standard treatment of RA follows a latter of severity depending on the stage at which the RA has progressed, in order; this includes physical therapy, medications, and surgery. Physical therapy is typically administered or suggested if the situation of RA is manageable, specifically if inflammationhas not caused severe deterioration of joint and/or bone. Surgical approaches are approached as a “last resort” treatment. Patients may choose to undergo joint replacement or arthrodesis. Patients with severe damage causes by RA, may choose to have joint replacements for large joints such as shoulders, hips, or knees as well as smaller joints in the fingers and toes. This surgical approach involves removing either all or part of a damaged joint and inserting a synthetic replacement. This procedure carries a costly and high health risk consequence [34]. The pain experienced by patients with severe or end-stage RA may outweigh the side effects, cost, and health risks associated with both surgical approaches in expectations to alleviate pain and swelling. The current pharmaceutical treatment options for RA also depend on stage of progression a patient is experiencing. Treatment is best administered in early stages of RA to reduce the likelihood of progression and destruction of synovial joints [35]. However, the clinical symptoms associated with early stage RA are often overlooked and/or mistaken for other arthritic diseases (such as OA). Patients with RA will typically seek medical attention when noticeable pain and swelling become overwhelming in daily activities. These symptoms are often indicators of moderate stage RA or the sequential stages. The administration of long-term treatment medications is generally delivered to patients in moderate stage RA and beyond. These include a class of drugs called disease-modifying anti-rheumatic medications (DMARDs) such as methotrexate, hydroxychloroquine, and sulfasalazine, which aid in the suppression of inflammation [36].
Another treatment class of drugs called biologic response modifiers (biologics) are administered to suppressboth cell-mediated and humoral response adaptive immune cells associated with the disease as well as blocking various chemokines. Examples of some of the biologics used in the treatment of RA include adalimumab (Humira), etanercept (Enbrel), and infliximab (Remicade), which block chemokines like tumor necrosis factor. Other biologics include abatacept (Orencia) that suppress T cell functions and rituximab (Rituxan), which suppresses B cell activity [37]. Other drugs include NSAIDs can be used to treat acute flares of inflammation in RA patients by suppressing inflammation and reducing pain such as ibuprofen, but these medications do not reduce joint damage or progression of RA. Additionally, glucocorticoids are strong anti-inflammatory steroids that may relieve symptoms but should only be taken in a short period of time due observed side effects. The notable trend among the varying medications is to relieve pain, swelling, and inflammation by suppressing the functions of the body’s immune system[39,40]. Although there has been great success in relieving pain in RA patients, there are major downsides to these treatment options. None of the medications possess the capability to fully stop the progression of the disease or regenerate tissue to replace the damaged areas. Also, there are a variety of adverse side effects associated with drugs that suppress the immune system due to patient susceptibility to infections that normally would not occur.Stem cells hold a unique capacity to modulate immune responses and regenerate lost tissue. The promising advantages of stem cell therapy in the treatment of RA hold the potential of stopping the progression of RA, regenerating damaged joint tissues, and relieving symptoms while avoiding immune rejection and maintaining immune regulation [41].
Discussion
Stem cells: an overview
Stem cells are the body’s master cells and offer great potential in the field of medicine. These cells can develop into many cell types of the body. Stem cells are undifferentiated cells that retain the ability of self-renewal and give rise to specialized cells that can be capable of replacing old or lost cells [42]. Stem cell therapy is the next chapter in the medical field with hopes to improve or replace organ transplantation, understanding the pathology and etiology of diseases, testing the safety and effectiveness of new drug treatments, and much more. For many years, researchers have been seeking further understanding and efficient methods to harness the unique capability of stem cells. Stem cells can be categorized into two groups, embryonic and adult. Embryonic stem cells are harvested from embryos following in vitro fertilization. The process of in vitro fertilization involves placing an oocyte and sperm together in a culture dish to allow fertilization [43]. Human embryonic stem cells (nESCs) are undifferentiated cells that are derived from the inner cell mass (ICM) of a blastocyst. The ICM cells hold the potential to generate all cell types of the human body. hESCs are obtained by harvesting a blastocyst and extracting the ICM. The ICM can then be cultured on appropriate medium to produce germ layers. There are three germ layers known as the ectoderm, mesoderm, and endoderm. Each of these layers give rise to their respective tissues and organs of the body [44]. The differential capacity of hESCs holds great promise to regenerative medicine and clinical treatment; however strong opposition of the isolation and use of hESC persists due to ethical concerns. Adult stem cells are another type of stem cell category. Adult stem cells, unlike hESCs, are multipotent, therefore are restricted in their differential capabilities. However, it is arguable that adult stem cells hold an equal or greater potential in a clinical setting than hESCs. ASCs were once thought to be in a rare and limited amount in the human body. In the last decade, research and studies have surged in identifying and discovering ASCs in a variety of tissues [45]. Adult stem cells can be found throughout almost the entire human body after development such as bone marrow, blood, umbilical cord tissue, umbilical cord blood, amniotic fluid, urine, skin, dental pulp, adipose tissue, etc. Adult stem cells hold a particular interest over hESCs because of the lack of ethical concerns regarding the harvesting of these cells [46].
Mesenchymal Stem Cells
Mesenchymal stem cells (MSCs) are a non-hematopoietic stem cell capable of multipotent differentiation. Of the various types of MSCs, bone marrow MSCs (BM-MSCs), adipose tissue MSCs (ADSCs), and umbilical cord tissue MSCs (UC-MSCs) are well known sites of abundant populations of MSCs [47]. Initially, researchers investigated and tested MSCs based on tissue repair and as a potential tool in regenerative medicine. As MSCs studies continued, it was discovered that MSCs have unique immunological properties making them viable candidates for the treatment of autoimmune disorders, such as RA. These properties include inflammatory, immunoregulatory, and immunosuppressive capacities, which contribute to MSCs as immune tolerant agents. The observed immunosuppressive effects of MSCs assist in overcoming immune rejection and allogenic transplantation barriers [48]. Despite the various tissue sources, a minimal set of criteria was proposed and is widely accepted by the Mesenchymal and Tissue Stem Cell Committee of the International Society for Cellular Therapy in 2006 [49]. This includes that human MSCs are defined as cells with the ability to be plastic-adherent culture conditions, expression of cell surface markers and express multipotent cell differentiation potential in vitro. The minimal criteria for cell surface marker expression, specially cluster differentiation, include CD73, CD90 and CD105, and unexpressed markers include CD11b, CD14, CD19, CD34, CD45, CD79a and HLA-DR [50, 51, 52, 53]. Establishing criteria for defining MSCs set the stage for scientists to pursue the successful identification and presence of MSCs in human adipose tissue, umbilical cord, bone marrow, amniotic fluid, and dental pulp, amongst other sources. Upon the finding of MSCs in various tissues, studies are underway and have been reported in further classifying MSCs based on cell surface marker expressions specific to different tissue sources. Efficient populations of MSCs have been reported in adipose tissue, bone marrow, and umbilical cord tissue. Of interest in the present review, the three sources of MSCs previously mentioned are of particular interest in treating Rheumatoid Arthritis.
Adipose tissue was demonstrated as a rich source of MSCs in 2001 [54]. Adipose tissue is the anatomical term given to tissue commonly known as fat tissue. After the discovery of these cells, various terminologies were proposed such as processed lipoaspirates, adipose-derived adult stem cells, adipose mesenchymal stem cells, adipose-derived stromal/stem cells, and adipose stromal cells. Due to the increased interest of these cells and publications, the International Federation of Adipose Therapeutics (IFATS) adopted the ADSCs nomenclature to limited confusion and ensure uniformity [55]. The extraction of adipose MSCs is a less invasive process than that of bone marrow MSCs. Surgical procedures, such as liposuction, is an indirect method for obtaining large amounts of adipose tissue necessary to harvest adipose MSCs. The most common and effective procedure for isolating ADSCs from fat tissue consists of using collagenase digestion, followed by centrifugal density gradient separation [56]. Other studies have been reported that in addition to collagenase, adipose tissue digested by dipase, trypsin and other related enzymes have been shown to be effective. The subcutaneous adipose tissue which is the source of ADSCs consists largely of mature adipocytes and a heterogenous stromal vascular fraction (SVF). This heterogeneous mixture consists of mesenchymal population of cells that includes adipose stomal, hematopoietic stem, hematopoietic progenitor cells, endothelial cells, erythrocytes, fibroblasts, lymphocytes,macrophages, and pericytesothers[57]. Importantly, ADSCs are distinguished from adipocytes due to their spindle-like morphology and lack of intracellular lipid droplets which are seen in adipocytes. ADSCs are also selected byadhesion properties in culture from SVF cells. When SVF cells are harvested, isolated and seeded into culture there is an observed subset of elongated cells that adhere to the plastic ware of the culture dish are what are known as the adipose derived mesenchymal stem cells [58]. The isolated ADSCs can be further purified using a combination of washing steps and culture expansion. The media used to expand ADSCs in vitro has been reported to be similar to that of bone marrow derived MSCs. The primary culture medium for MSCs includes low-glucose Dulbecco’s modified Eagle medium (LG-DMEM) with 1% (W/V) antibiotic/antimycotic and 10% (V/V) fetal bovine serum (FBS) [59]. In the literature, numerous studies strongly implicate that ADSCs possess a variety of differentiation potential in vitro including adipogenic, osteogenic, chondrogenic, myogenic, cardiogenic, and neurogenic differentiation. This ability to differentiate into these cell types along with the ease of an abundant and less invasive harvest method make ADSCs highly considerable as tools for replacing, repairing, and regenerating dead or damage cells. For the purpose of this study, ADSCs ability to differentiate into osteogenic and chondrogenic cells make ADSCs possible for stem cell therapy forRA patients with articular cartilage and bone destruction. It has been noted in various studies that ADSCs proliferation rate is affected by age, unlike their differentiation capability. Specifically, it has been reported that cells from younger donors exhibited a higher proliferation rate when compared to elderly ones. However, the differentiation capacity was maintained with aging [60]. Another unique quality that further validates ADSCs as a better suited therapeutic candidate for Rheumatoid Arthritis than current treatment medications is the observed immunomodulatory capabilities of ADSCs. Specifically, ADSCs have a hypoimmunogenic phenotype resulting from the absence of the MHC class II proteins and low expression levels of MHC class I, this allowing them to evade immune recognition without the need for immune suppression. Furthermore, the observed immunomodulating effects of ADSCs in the literature is linked to higher levels of cytokine production [61]. The precise mechanisms that enable ADSCs to modulate immune recognition is not fully understand because of the limitations of valid results gathered from in vitro analysis. However, pre-clinical trials and clinical trials have implicated that these mechanisms are rely on a coupled blend of cell-to-cell contact mechanisms and paracrine effects through the production of cytokines and various soluble factors[62].Paracrine factors (or growth and differentiation factors- GDFs) refer to the proteins synthesized by one cell that can diffuse across small distances to induce changes in neighboring cells through a paracrine interaction [63]. These regulate immune cells functions, stimulate the microenvironment for tissue healing, and exert immunosuppressive effects by decreasing inflammatory cytokine production. These findings contribute to making ADSCs a viable option in regenerative medicine and aneffective tool in cell-based therapy for restoring damaged tissues. As for RA, stem cell therapy using ADSCs holds great potential to decrease inflammatory and immune responses, which has an advantage over anti-inflammatory steroids due to the ability to provide a reduction in inflammation without suppressing the entire immune system. One immunomodulating property of ADSCs that has struck interest regarding regenerative medicine and autoimmune diseases is their ability to inhibit proliferation of pro-inflammatory T cells [64].
Bone marrow is source of two types of stem cells, hematopoietic stem cells (HSCs) and mesenchymal stem cells (MSCs). The discovery of hematopoietic stem cells in bone marrow was first made by investigators Till and McCulloch in 1961[66]. Although controversy amongst scientist remains on the first valid discovery of stem cells, it is widely accepted that the first discovery and identification of the presence and basic properties of stem cells are credited to Ernest McCulloch and James Till. Following the atomic bomb explosions on Hiroshima and Nagasaki 1945, scientists sought to understand the effects of radiation on the civilians and soldiers that were exposed. Specifically, it was observed that full body irradiation compromised the hematopoietic system of the body hindering its function in the production of sufficient amounts of crucial blood elements that function to fight infections, form blood clots, etc. In the 1950s, the initial sighting of recovery from whole-body irradiation was observed in irradiated mouse models in which the injection of suspensions of cells from blood-forming organs such as the bone marrow recused the otherwise hematopoietic system failure [65]. In 1956, E. Donnall Thomas conducted the first successful bone marrow transplantation on identical twins effected by total body irradiation. However, it was not until the radiation physicist, James Till, joined arms with the leukemia specialist and hematologist, Ernest McCulloch, that made the astounding discovery of hematopoietic stem cells while conducting a study on the radiation effects on mice. The work of McCulloch and Till laid the foundation for modern attempts to isolate, study, and use stem cells for medical purposes [66]. During the late 1960’s, Alexander Friedenstein and colleagues were of the first to pioneer the theory and discovery of another type of stem cell present in bone marrow, mesenchymal stem cells[67]. Friedenstein and colleagues observed the presence of MSCs when culturing cells isolated from the bone marrow of rats. During the progression of their studies, it was noted that when BM cells were seeded in a flask and maintained in appropriate culture media there was a production of non-hematopoietic cells that were morphologically similar to fibroblasts and adhered to the plastic of the culture flask. Furthermore, these cells formed colonies that were transplantable. These transplantable colonies were demonstrated by this investigator to be capable of self-renewal and also form mature nonhematopoietic tissues in recipient animals, such as bone, cartilage, and fibrous tissue thus indicating their potential in stem cell nature and tissue regeneration. In the 1970s and 1980s, more studies were conducted that extended the observations made by Friedenstein further verifying that the non-hematopoietic cells in bone marrow are multipotent and could give rise to osteoblasts, chondrocytes, and adipocytes.
Umbilical cord tissue is another source of mesenchymal stem cell. The umbilical cord forms from the yolk sac and allantois. During embryonic development, the umbilical cord becomes a channel structure between the developing embryo and the placenta. The umbilical cord stroma contains viscous substance called Wharton’s jelly (WJ). This is named after an English physician and anatomist [68]. In 1956, Thomas Wharton is credited to the first description of Wharton’s jelly (WJ). In the1970’s, the umbilical cord blood was recognized as a source of HSCs, but the remaining umbilical cord tissue was considered medical waste with no scientific value and was discarded. A change to this concept occurred in 1991, when McElreavey became the first to isolate and characterize the fibroblast-like cells from the WJ portion, or tissue portion, of the umbilical cord [69]. The umbilical cord, what once was discarded as medical waste after-birth, is now a noted as a prime source of MSCs. After birth, the umbilical cord may be stored as a source of stem cells genetically identical to the child. Thus, if the child is diagnosed with a specific condition that seeks treatment, then the MSCs within the stored umbilical cord may be used for transplant avoiding the risk of immune rejection. Secondly, the mother has the option to donate the umbilical cord for medical research and use following informed consent [70]. UC-MSCs are globally accessible tissues with little to no ethical concerns since they were once treating as medical waste. UC-MSCs collection method has an advantage over BM-MSCs and ADSCs due to the lack of invasive procedures required to obtain the cells. Several experiments have been conducted to validate the cells in question as MSCs based on the minimum criteria (1) adherence to bottom of flask in culture (2) expression of CD73, CD90 and CD105, and unexpressed markers include CD11b, CD14, CD19, CD34, CD45, CD79a and HLA-DR and (3) differentiation into osteoblasts, chondroblasts and adipocytes. Moreover, combined with the well-documented self-renewal and multipotent differentiation potentials, UC-MSCs possess immunoregulatory capacities that contribute to the potential for treatment of RA patients.
Comparative studies of MSCs derived from umbilical cord tissue, bone marrow, and adipose tissue
Mesenchymal stem cells (MSCs) hold great potential in the medical field over other sources of stem cells. However, there is a substantial amount of information regarding the mechanisms and efficacy of MSCs that is unknown. Many researchers and scientists in pursuit of further understanding of MSCs have conducted comparative studies amongst MSCs derived from different sources. The majority of the studies focus on the abundant populations of MSCs present in bone marrow, adipose tissue, and umbilical cord tissue [71]. Moreover, comparative analyses and experiments have and are currently investigating functional characteristics of MSCs crucial for cellular therapy such as proliferation capacities, differentiation capacities, migratory properties, immunomodulating/inflammatory functions, and others. MSC origin may be an important factor in determining biological activity and therapeutic use.
BM-MSCs harvesting is among the most invasive and painful procedures involving general anesthesia and hospital stay for medical attention/observation. The quantity of BM obtained from the procedure is dependent on the patient status and the volume of aspirates. These aspects combined with the extremely low presence of BM-MSC population within bone marrow, place BM-MSCs at a disadvantage to other sources of MSCs [72]. However, BM-MSCs are known as the “gold standard” when comparing MSCs from various human tissues in respect to their historical and deeper clinical understanding. The extensive research and analysis conducted using BM-MSCs have provided clues to understanding the proliferation, differentiation, culture, and immune regulation capacities along with effective testing strategies.Lee et al. reported that when examined in culture expansion, BM-MSCs stopped growing by passage 11–12 and highly expressed the senescence-related proteins p53, p21, and p16 [73]. Lee et al. Additionally, BM-MSCs had a lower activity of inflammatory regulation than in ADSCs with 24 genes associated with immunomodulation that were down regulated in BM-MSCs when compared to ADSCs [73].Another study conducted a comparative examination based on gene expression of BM-MSCs, ADSCs, and UC-MSCs [74]. The results concur with the minimum criteria of MSCs amongst the mentioned MSCs. It was also noted that all MSCs were phenotypic similar and of fibroblast morphology. UC-MSCs in vitro reported having a higher proliferative capacity than BM-MSCs and ADSCs. Among these studies, the numerous findings compliment each other with similar conclusions regarding the lack of significant differences among morphology, self-renewal capacity, and fibroblast growth. Consequently, more knowledge on the different abilities of MSCs is mandatory toovercome obstacles influencing increase large-scale and viable production of MSCs for therapeutic use. The shortage of evidence regarding the matter is a possible consequence of the lack of standardized protocols for expanding MSC cultures in vitro.
The differentiation potential of stem cells is an important trait in determining which cell may be more effective than others in treating clinical conditions in question. Insights to the underlying mechanisms of how and when MSCs commit to specific lineages enable scientists to develop techniques to manipulate the cells for clinical applications. As discussed, the ability to differentiate into adipogenic, chondrogenic, and osteogenic lineages is one of thecriteria for MSCs. Differentiation of MSCs in the appropriate cell type is assessed by identifying the production of respectively: fat droplets (adipogenic), proteoglycans and type II collagen synthesis (chondrogenic) or mineralization of calcium deposits and the increase of alkaline phosphatase expression (osteogenic)[74]. This differentiation activity can be analyzed in vitro by placing MSCs in a culture medium with special supplements [75]. The addition of these supplements into the medium is a valuable tool in directed the differentiation of MSCs into specific lineages and to access efficiency. The adipogenesis process generally consists of adding dexamethasone, indomethacin, insulin and isobutylmethylxanthin. For chondrogenic differentiation, cell culture in DMEM medium (Dulbecco / Vogt Modified Eagle’s Minimal Essential Medium) supplemented with insulin, transferrin, selenium, linoleic acid, selenium acid, pyruvate, ascorbic phosphate, dexamethasone and TGF-β III, which may additionally be aided by the addition of IGF-1 and BMP-2. Moreover, the osteogenesis is induced by the presence of ascorbic acid, β-glycerophosphate and dexamethasone in medium. The literature reports proposed and identified that supplying other appropriate elements in medium could induce MSCs to be a source of other cell types [76]. Indeed, it has been widely reported that in addition to mesodermal lineage MSCs have ectodermal and endodermal lineage differentiation potential in strictly appropriate environment. Xuet al., reported significant osteogenesis and adipogenesis differentiation abilities of ADSCs and BM-MSCs in mouse models [77]. Osteogenesis differentiation potential was assessed by the mRNA expression levels of genes related to osteogenesis were detected by quantitative real-time RT-PCR (qRT-PCR). The data implicated that BM-MSCs possessed a slightly higher potential for differentiation into osteoblasts than ADMSCs. In the same manner of osteogenesis differentiation assessment, qRT-PCR was used to examine the mRNA expression levels of genes related to adipogenesis. The data displayed a higher adipogenic differentiation potential of ADSCs than BM-MSCs. However, it was noted that there was no significant difference in chondrogenic differentiation amongst the two. Further findings of this study suggest that the differentiation potential of MSCs is highly influenced by their tissue of origin through epigenetic regulations, such as DNA methylation of important transcription factors.
One of the many beneficial of mesenchymal stem cells is their immunomodulation capacity and paracrine factors, making MSCs considerable for inflammatory and immune-mediated diseases. Immunomodulatory functions have been reported for all types of MSC tested [78-80]. Additionally, these unique attributes led to the introduction and administration of MSCs as potential therapeutic tools to correct the breakdown of autoimmunity in RA, particularly for patients that are inadequately treated or resistant to current treatments. MSCs are of newly found interest in the treatment of immune related disorder. For the purpose of this study, the immunological properties of MSCs will be discussed in regard to the possible treatment of RA. The imbalance between the immune response of pro-inflammatory and anti-inflammatory cells, in particular between memory Th17 and memory regulatory T cells is widely observed in the literature to be linked to RA immunopathogenesis [81]. In consequence to the complex mechanisms involved with the propagation of RA as well as MSC immunosuppressive functions, it is imperative to examine the potential immunological functions of MSCs in immune elements of RA in order to provide better MSC-therapy and clarification of the pathogenesis of RA. Even with the substantial advances that have been made in the generation of novel therapies and medications against RA, there are still a lot of patients that are resistant to treatment. Hence, it is reasonably proposed to think that the resistance of memory T cells could be a main contributor to the absence of a beneficial response of these immunomodulatory therapies and medications [82]. Mesenchymal stem cells can be used to adjust autoimmune responses and in autoimmune disease treatment because they cause immunosuppression by modulating T and B cell proliferation and differentiation, dendritic cell growth and the activity of natural killer cells. Much of the MSC literature has placed the anti‐inflammatory and immunomodulatory properties of MSCs front and center in much of the recently published journals due to the widely observed effects among various experiments, culture conditions, and combination treatments with other pharmaceuticals. Specifically, MSCs respond to their immediate environment and adjust their response accordingly through the release of soluble factors such as prostaglandin E2 (PGE2), kynurenine, interleukin (IL‐10), tumor necrosis factor (TNF)‐stimulated gene 6 protein (TSG‐6), nitric oxide (NO), and transforming growth factor (TGF‐β)‐1 [83,84]. Using human cells in vitro, Quaedackers et al., demonstrated that activated CD8+ and CD4+ T‐cells were specifically modulated through binding to adipose‐derived MSCs [85]. These results implicate that the binding of cell-mediated immune cells to ADSCs represents an immunomodulatory mechanism in which CD8+ T cells are inhibited in their responsiveness to pro-inflammatory stimuli and effector CD4+ T cells are depleted from the immune response. As discussed previously, the current understandings of the pathology of RA could be combatted by the immunomodulation effects of MSCs.BM-MSCs were able to decrease the proliferation of memory T cells (CD4+CD45RO+). In addition to the immunomodulation of the proliferation of memory T cells, another noteworthy therapeutic effect of MSCs is their capacity to migrate and home into inflamed tissues. MSCs, specifically BM-MSCs, have a variety of expressed chemokine receptors involved in MSCs migration [86,87]. Thus, such MSCs could potentially migrate into the inflamed synovial membrane of RA patients and interact with memory T cells, inhibit their proliferation rate or/and alter their pro-inflammatory cytokine secretionthusresulting in a reduction of inflammation in the synovial membrane. This exhibits a potentially better treatment of RA with the use of MSCs through their cell-to-cell interactions and immunomodulatory mechanisms than the present pharmaceuticals used.
Pre-Clinical Trials
Due to the ethical and technical limitations on examining MSCs in humans, animal models are used to test new pharmaceuticals and cell-based therapies. Furthermore, they are used to model certain autoimmune diseases, such as RA to better our understanding of the progression of the disease. The need for animal models must include accurate representation of the pathogenesis of the autoimmune disease in question and allow pre-clinical testing of cell-based therapeutic approaches targeting human cells and tissues in vivo [88]. The conventional animal models currently used represent classic hallmarks of RA, such as joint swelling, synovitis, pannus formation, and bone erosion. However, the conventional models differ in the mechanisms of induction of immune processes, as well as in their speed of onset, chronicity, and severity. Two mouse models of have been used in a wide variety of examinations of RA, which are the induced and spontaneous mouse models [89]. In induced models, non-specific immune activation, cartilage-directed autoimmunity, or abundant exogeneous (environmental) factors cause the mirror representation of RA pathogenesis. In spontaneous models, arthritis develops without deliberate immunization and is non-limiting, providing a chronic/progressive situation like in human RA. Collagen-induced arthritis (CIA) is the most commonly used model of RA-like disease. In this model, severe joint inflammation of the model is induced through injection with Type II Collagen, a major component of hyaline cartilage found in the articular capsule, together with CFA. Important limitations when considering CIA is that it is an acute model, meaning remission occurs at 10–14 days after disease onset (unlike humans), extra-articular manifestations are due to CFA, and disease severity is highly variable and dependent on environmental factors, such as grouping stress[90]. Another limitation that is considered is that joint inflammation in CIA mice is exceptionally mediated by Th17 cells, while the pathogenesis of human RA involves Th1, Th17, Th1/17, and various other immune cellular pathways. Limitations regarding mouse models must be considered in cross-examining treatments in the comparison to humans. A common weakness of all conventional models of RA is that immune responses are completely mediated by the murine immune system found in mice, which differs in its components and organization from the human immune system [91]. This comes as no surprise that many successful results observed in mouse models are not observed in humans. However, scientists and researchers have overcome this species-species barrier by creating humanized models of mice.This humanization strategy is based on transgenic expression of human molecules, such as MHC class II proteins, RA-associated synovial autoantigens and/or an autoantigen-specific T cell receptor, in immunocompetent mice[92]. This is greatly advanced the understanding the pathogenesis of RA as well as other autoimmune diseases.The phrase “bench-to-bedside” is given to the method of experimentingin pre-clinical trials with the goal of translating to clinical treatment. To date, there have been numerous MSC-based pre-clinical trials, which is further discussed highlighting a few of the major findings based on MSC treatment of RA.
In 2015, a study was conducted to compare the effectiveness of BM-MSCs and hematopoietic stem cells (HSCs) from human umbilical cord blood in the treatment of RA in rat models [93]. The study was carried out on 40 male Wistar rats that were divided into 5 groups each consisting of 8 animals. Group 1, the negative control group, received 1 mL saline by intravenous injection. Group 2, the positive control group, received an injection of 0.1mL of complete Freund’s adjuvant (CFA) to induce arthritis, which produced definite edema within 24 hours in addition to progressive arthritis by the 9th day after inoculation. Group 3 (MTX) comprised of arthritic induced rats that received 2 mg/kg of methotrexate (MTX) once a week for 4 weeks. Group 4 (MSC) consisted of arthritis rats that received MSCs in the form of a cell suspension in a single intravenous dose containing 1x106 allogeneic MSCs derived from the bone marrow of rats. Group 5 (HSC) consisted of arthritis rats that received HSCs in a single intravenous dose in the form of a cell suspension containing 1x106 allogeneic HSCs derived from human umbilical cord blood (UCB). It was reported that the group injected with MSCs resulted in the highest reduction in RA inflammation. Specifically in this study it was noted that the rapid reversal of tissue inflammation observed in Group 4 (MSC) was accompanied by a diminished tissue level of IL-6, TNF-a, TGF-b, NF-KB, TLR-2, MMP-3, COMP-1, and RF, a better antioxidant state and an elevated production of anti-inflammatory cytokine IL-10. This concurs with other studies that show the ability of MSCs to suppress immune system components in RA pathogenesis.
Another study investigated and compared the therapeutic effects of MSCs derived from human bone marrow, adipose tissue, and umbilical cord tissue in collagen-induced arthritic (CIA) mice [94]. Several factors were examined in this study, (a) the optimal number of MSCs injected and the treatment regimen for the CIA model, (b) to explore the mechanism underlying immune modulation by MSCs in CIA, and (c) the effectiveness of MSCs in clinical arthritis scores, synovial inflammation, and cartilage and bone erosion in CIA mice. The results of the experiment concur with other research and literature studies, implicating promising translation potential of MSC-based therapy for RA. Additionally, a thorough assessment was conducted in this particular study amongst the effectiveness of each MSC tested.It was demonstrated that BM-MSCs had a greater therapeutic efficacy than ADSCs or umbilical cord blood-MSCs in CIA, concluded fromexamination of the severity of arthritis and histopathological evaluation. For the immunohistochemical analysis of the study, it was noted that IL-1b, IL-6, and TNF-a were expressed less amounts in BM-MSC- and ADSC-treated mice than in umbilical cord blood-MSCtreated mice. Among the three types of MSCs, there were similarities in the amount of proinflammatory cytokines and anti-inflammatory cytokines. The number of memory T cellswere higher in the BM-MSC treated mice than in the ADSC- and umbilical cord blood-MSC-treated mice.The memory T cell suppression assay conducted in the studyimplicated that memory T cells induced by BM-MSCs had stronger immunosuppressive activity than those induced by ADSCs or umbilical cord blood-MSCs. Notably, the study concluded from data thatmemory T cells induced by MSC have more immunomodulation activity than those of untreated CIA mice. Also, the human MSCs examined were purposely used in MHC-mismatch mice for the evaluation. This was done to examine the effectiveness of reducing immune system recognition which is crucial in administering MSCs. The data suggested that MSCs were not rejected by the immune system, even after allogenic or xenogenic transplantation, because T cells do not recognize them because MSCs lack class II MHC proteins. This implies that allogenic MSCs might be a functional and effective delivery method for stem cell therapy in clinical settings.
Clinical Trials
In this context, according to the U.S National Library of Medicine clinical trial database (https://clinicaltrials.gov/), 15 MSC-based clinical trials for RA exist. Upon them, MSCs derived from bone marrow, umbilical cord, and adipose tissue are of high interest. In the present context, no adverse or severe side effects have been reported. The studies vary amongst investigations based on evaluating dose tolerance, comparing the efficiency of MSCs and current RA drug treatments, MSCs ability to alleviate/stop inflammation associated with RA, MSCs ability to control other immune responses associated with the propagation of RA, and evaluating the safety of allogenic MSC transplantation. From the results examined of the completed trials, scientists and researchers have determined a consistency of the safety and effectiveness of BM-MSCs, UC-MSCs, and ADSCs in the transplantation and treatment of RA [95,96]. In one study conducted in a phase Ib/IIa clinical trial, the intravenous administration of allogeneic ADSCs in a study comprised of 53 patients with a placebo group was shown to be safe and well tolerated in refractory RA patients [97,98]. Unfortunately, among the other clinical trials, the results are partial and temporary because of short term follow-up. With a vast of information necessary in understanding the mechanisms by which MSCs wield their therapeutic function in RA, more clinical trials with large sample sizes and mandatory follow up protocols will yield information that will surely help in the pursuit of MSCs for stem cell therapy.
A highlighted study in 2019 was conducted by Huang et. al., reporting the long-term efficacy of treating RA patients with MSCs derived from umbilical cord tissue [99]. The data collected from the 3-year cohort study demonstrated that UC-MSC treatment was safe and able to benefit RA patients in long term when combined with DMARDs. The experiment preceded by inclusion and exclusion guidelines regarding the test subjects. The eligibility requirements consisted of swelling that is present in at least one joint, and three or more joint swelling; morning stiffness for 3 hrs on average, which did not have another cause; results from at least one blood test indicate the presence of RA; symptoms have been present for at least 6 weeks. Patients were rendered as ineligible to the study if pregnant, aged under 12 or over 70 years, and/or had received any RA treatment before. All patients’ blood samples were collected before treatment, 1 year, and 3 years after UC-MSC treatment. Safety assessments were performed by testing blood routine of liver and kidney functions and immunoglobulin levels, stability assessment by HAQ and DAS28 and serological markers included RFand ACPAs. The noteworthy design of this study is arguably one of the best proposed methods for determining the effectiveness of MSC-therapy. Furthermore, the long-term results of the study are considerably promising. Data was collected 1-3 years post-treatment to access safety, changes of inflammatory and serological markers, and overall efficacy. The data collected from the long-term safety evaluation demonstrated liver, kidney function and immunoglobulins levels from each patient were all within normal range before 1 year and 3 years after UC-MSC treatment. Moreover, there were significant decreases for the Inflammatory and/or RA serological makers including RF and ACPAs observed during the posttreatment evaluation. Lastly, detailed data sets were collected of two individual patients. A 68-year-old man who had serious joint pain, swelling and deformity before treatment had achieved remission for 6 months after UC-MSC treatment and maintained till 3 years after treatment. This patient made a remarkable recovery.The joint deformity caused by RA recovered and the pain diminished. The astonishing recovery of joint function was also observed in the second individual of interest, a 33-year-old woman with severe joint swelling and deformity.
Conclusion and Future Viewpoints
Memory T cells and genetic factors have been largely studied for their key role in the immunopathogenesis of autoimmune disease such as RA. MSCs are multipotent cells with broad immunomodulatory properties and therefore have been proposed as a prime candidate of choice for autoimmune diseases treatment due to their ability to suppress the activity of pathogenic T cells without suppressing the overall functions of the immune system. Pre-clinical trials and clinical trials have shown strong therapeutic potential for MSC-based therapy for RA. However, amongst the positive results remains inconsistency in respect to the most effective delivery method, dosage and source of MSCs. Thus, it is necessary to increase the number of patients and studies in order to draw fundamental conclusions regarding MSC therapeutic effects in RA. Furthermore, it must be considered that amongst clinical trials discussed today are patients with severe RA. MSC treatment in early stages of RA could demonstrate improved results as well as increase the understanding of the root cause of RA.A crucial innovation in determining the efficiency of MSCs infusion for RA treatment is to develop a detailed immune-monitoring analysis method of RA patients that examines the dynamics of both pathogenic and non-pathogenic memory T cells upon stem cell treatment. Future advancements in identifying the full-scope of the pathology of RA by using stem cell therapy holds great potential in understanding a wide variety of factors causing rheumatological diseases and cancer that carry a massive unresolves burden worldwide.
References
- Lin YJ, Anzaghe M, Schülke S. (2020) Update on the Pathomechanism, Diagnosis, and Treatment Options for Rheumatoid Arthritis. Cells. 9(4):880.
- Fugger L, Svejgaard A. (2000) Association of MHC and rheumatoid arthritis: HLA-DR4 and rheumatoid arthritis-studies in mice and men. Arthritis Res Ther. 2(3):1-5.
- https://www.medicinenet.com/rheumatoid_arthritis/article.html
- Hitchon CA, El-Gabalawy HS. (2011) Suppl 1: the synovium in rheumatoid arthritis. Open Rheumatol J. 5:107.
- Sokka T, Toloza S, Cutolo M, Kautiainen H, Makinen H, et al. (2009) “Women, men, and rheumatoid arthritis: analyses of disease activity, disease characteristics, and treatments in the QUEST-RA study.” Arthritis Res Ther. 11(1): R7.
- https://curearthritis.org/arthritis-archives-rheumatoid-arthritis/
- https://www.webmd.com/rheumatoid-arthritis/ra-and-women
- Dowdy SW, Dwyer KA, Smith CA, Wallston KA. (1996) Gender and psychological well‐being of persons with rheumatoid arthritis. A&R. 9:449-56.
- Sierakowski S, Cutolo M. (2011) Morning symptoms in rheumatoid arthritis: a defining characteristic and marker of active disease. Scand J Rheumatol Suppl. 125:1-5.
- Yap HY, Tee SZ, Wong MM, Chow SK, Peh SC, et al. (2018) Pathogenic role of immune cells in rheumatoid arthritis: implications in clinical treatment and biomarker development. Cells. 7(10):161.
- Elshabrawy HA, Chen Z, Volin MV, Ravella S, Virupannavar S, et al. (2015) The pathogenic role of angiogenesis in rheumatoid arthritis. Angiogenesis. 18(4):433-48.
- Bartok, Beatrix, and Gary S Firestein. (2010) “Fibroblast-like synoviocytes: key effector cells in rheumatoid arthritis.” Immunol Rev. 233(1):233-55.
- Zhang JM, An J. (2007) Cytokines, inflammation, and pain. IntAnesthesClin. 45(2):27-37.
- Xu B, Jin L. (2017) “Characteristics and risk factors of rheumatoid arthritis in the United States: an NHANES analysis.” Peer J. 5:e4035.
- Sonnenberg, Gregory F, Matthew R Hepworth. (2019) “Functional interactions between innate lymphoid cells and adaptive immunity.” Nat Rev Immunol. 19(10):599-613.
- Clénet ML, Gagnon F, Moratalla AC, Viel EC, Arbour N. (2017) Peripheral human CD4+ CD8+ T lymphocytes exhibit a memory phenotype and enhanced responses to IL-2, IL-7 and IL-15. Sci. Rep. 7(1):1-5.
- Chalan P, Kroesen B, Geest K, Huitema M, Abdulahad W, et al. (2013) “Circulating CD4+CD161+ T lymphocytes are increased in seropositive arthralgia patients but decreased in patients with newly diagnosed rheumatoid arthritis.” PloS one. 8(11):e79370.
- Schütz C, Oelke M, Schneck J, Mackensen A, Fleck M. (2010) “Killer artificial antigen-presenting cells: the synthetic embodiment of a 'guided missile'.” Immunother. 2(4):539-50.
- Taneja V, David CS. (2000) “Association of MHC and rheumatoid arthritis. Regulatory role of HLA class II molecules in animal models of RA: studies on transgenic/knockout mice.” Arthritis Res. 2(3):205-7.
- Alberts B, Johnson A, Lewis J, Raff M, Roberts K, et al. (2002) Molecular Biology of the Cell. 4th Edn. New York: Garland Science. “T Cells and MHC Proteins.
- Duluc D, Banchereau R, Gannevat J, Thompson-Snipes L, Blanck J, et al. (2014) “Transcriptional fingerprints of antigen-presenting cell subsets in the human vaginal mucosa and skin reflect tissue-specific immune microenvironments.” Genome Med. (6)1198.
- Kim K, Bang S, Yoo D, Cho S, Choi C, et al. (2016) “Imputing Variants in HLA-DR Beta Genes Reveals That HLA-DRB1 Is Solely Associated with Rheumatoid Arthritis and Systemic Lupus Erythematosus.” PloSone.11(2):e0150283.
- Jin H, Arase N, Hirayasu K, Kohyama M, Suenaga T, et al. (2014) “Autoantibodies to IgG/HLA class II complexes are associated with rheumatoid arthritis susceptibility.” Proceedings of the National Academy of Sciences of the United States of America. 111(10):3787-92.
- Okada Y, Kim K, Han B, Pillai N, Ong R, et al. (2014) “Risk for ACPA-positive rheumatoid arthritis is driven by shared HLA amino acid polymorphisms in Asian and European populations.” Human molecular genetics. 23(25):6916-26.
- Ludwig R, Vanhoorelbeke K, Leypoldt F, Kaya Z, Beiber K, et al. (2017) “Mechanisms of Autoantibody-Induced Pathology.” Front Immunol. 8:603.
- Scally S, Law S, Ting Y, Heemst J, Sokolove J, et al. (2017) “Molecular basis for increased susceptibility of Indigenous North Americans to seropositive rheumatoid arthritis.” Annals Rheum Dis. 76(11):1915-23.
- Tan, Eng M, Josef SS. (2016) “Historical observations contributing insights on etiopathogenesis of rheumatoid arthritis and role of rheumatoid factor.” J Experiment Med. 213(10):1937-50.
- Burska A, Boissinot M, Ponchel F. (2014) “Cytokines as biomarkers in rheumatoid arthritis.” MediatInflam. 2014:545493.
- Huh Y, Lee G, Lee K, Koh J, Chun J, et al. (2015) “HIF-2α-induced chemokines stimulate motility of fibroblast-like synoviocytes and chondrocytes into the cartilage-pannus interface in experimental rheumatoid arthritis mouse models.” Arthritis Res Ther 17(302).
- Li H, Hong S, Qian J, Zheng Y, Yang J, et al. (2010) “Cross talk between the bone and immune systems: osteoclasts function as antigen-presenting cells and activate CD4+ and CD8+ T cells.” Blood Vol. 116(2):210-7.
- Meednu N, Zhang H, Owen T, Sun W, Wang V, et al. (2016) “Production of RANKL by Memory B Cells: A Link Between B Cells and Bone Erosion in Rheumatoid Arthritis.” Arthritis Rheumatol. 68(4):805-16.
- Abou-El-Hassan H, Hassan Z. (2017) “Viral-derived complement inhibitors: current status and potential role in immunomodulation.” Experimental biology and medicine (Maywood, N.J.) 242(4):397-410.
- https://www.cellmedicine.com/stem-cell-therapy-for-rheumatoid-arthritis/
- Jordan E, Gallicchio VS. (2020) Stem Cell Therapy as a Treatment Method for Rheumatoid Arthritis. Stem Cells Regen Med. 4(1):1-10.
- Bullock J, Rizvi S, Saleh A, Ahmed S, Do D, et al. (2018) “Rheumatoid Arthritis: A Brief Overview of the Treatment.” Medical principles and practice: international journal of the Kuwait University, Health Science Centre. 27(6):501-7.
- Gaitonde P, Bozzi L, Shaya F. (2018) “Factors associated with use of disease modifying agents for rheumatoid arthritis in the National Hospital and Ambulatory Medical Care Survey.” Sem Arthritis Rheumat. 47(5):649-53.
- Mysler E, Pineda C, Horiuchi T, Singh E, Mahgoub E, et al. (2016) “Clinical and regulatory perspectives on biosimilar therapies and intended copies of biologics in rheumatology.” Rheumatol Int. 36(5):613-25.
- Ringold S, Angeles-Han S, Beukelman T, Lovell D, Cuello C, et al. (2019) “American College of Rheumatology/Arthritis Foundation Guideline for the Treatment of Juvenile Idiopathic Arthritis: Therapeutic Approaches for Non-Systemic Polyarthritis, Sacroiliitis, and Enthesitis.” Arthritis Care Res. 71(6):717-734.
- England B, Thiele G, Anderson D, and Mikuls T. (2018) “Increased cardiovascular risk in rheumatoid arthritis: mechanisms and implications.” BMJ (Clinical research ed.) 361:k1036.
- Singh J, Hossain A, Ghogomu E, Mudano A, Maxwell L, et al. (2017) “Biologics or tofacitinib for people with rheumatoid arthritis unsuccessfully treated with biologics: a systematic review and network meta-analysis.” The Cochrane database of systematic reviews. 3(3):CD012591.
- JJ El-Jawhari, YM El-Sherbiny, EA Jones, D McGonagle. Mesenchymal stem cells, autoimmunity and rheumatoid arthritis. QJM. Int J Med. 107(7):505-14.
- Andrzejewska A, Lukomska B, Janowski M. (2019) Concise Review: Mesenchymal Stem Cells: From Roots to Boost. Stem cells. 37(7):855-64.
- Jafarzadeh H, Nazarian H, GhaffariNovin M, Shams Mofarahe Z, Eini F, et al. (2018) Improvement of oocyte in vitro maturation from mice with polycystic ovary syndrome by human mesenchymal stromal cell-conditioned media. J Cell Biochem. 119(12):10365-75.
- Kiecker C, Bates T, Bell E. (2016) Molecular specification of germ layers in vertebrate embryos. Cell Mol Life Sci. 73(5):923-47.
- Abu-Dawud R, Graffmann N, Ferber S, Wruck W, Adjaye J. (2018) Pluripotent stem cells: induction and self-renewal. Philos Trans R SocLond B Biol Sci. 373(1750):20170213.
- Gurusamy N, Alsayari A, Rajasingh S, Rajasingh J. (2018) Adult Stem Cells for Regenerative Therapy. ProgMolBiolTransl Sci. 160:1-22.
- Gargiulo C, Pham VH, Hai NT, Nguyen KCD, Van Phuc P, et al. (2015) Isolation and Characterization of Multipotent and Pluripotent Stem Cells from Human Peripheral Blood. Stem Cell Discov. 5:19-32.
- Luque-Campos N, Contreras-López RA, Paredes-Martínez MJ, Torres MJ, Bahraoui S, et al. Mesenchymal Stem Cells Improve Rheumatoid Arthritis Progression by Controlling Memory T Cell Response. Front Immunol. 10:798.
- Ullah I, Subbarao RB, Rho GJ. (2015) Human mesenchymal stem cells-current trends and future prospective. Biosci Rep. 35(2), e00191.
- Franceschetti T and De Bari C. (2017) The potential role of adult stem cells in the management of the rheumatic diseases. TherAdvMusculoskelet Dis. 9(7):165-79.
- Hofer, Heidi R, and Rocky S Tuan. (2016) “Secreted trophic factors of mesenchymal stem cells support neurovascular and musculoskeletal therapies.” Stem Cell Res Ther. 7(1):131.
- Karantalis, Vasileios, and Joshua M Hare. (2015) “Use of mesenchymal stem cells for therapy of cardiac disease.” Circulation Res. 116(8):1413-30.
- Fan X, Zeng Q, Li X, Li C, Xu Z, et al. “Induced pluripotent stem cell-derived mesenchymal stem cells activate quiescent T cells and elevate regulatory T cell response via NF-κB in allergic rhinitis patients.” Stem cell Res Ther. 9(1):170.
- Han Y, Li X, Zhang Y, Han Y, Chang F, et al. (2019) Mesenchymal Stem Cells for Regenerative Medicine. Cells. 8:886.
- Kocan B, Maziarz A, Tabarkiewicz J, Ochiya T, Banaś-Zabczyk A. (2017) “Trophic Activity and Phenotype of Adipose Tissue-Derived Mesenchymal Stem Cells as a Background of Their Regenerative Potential.” Stem cells Int. 2017:1653254.
- Ceccarelli S, Pontecorvi P, Anastasiadou E, Napoli C, Marchese C. (2020) Immunomodulatory Effect of Adipose-Derived Stem Cells: The Cutting Edge of Clinical Application. Front Cell Dev Biol. 8:236.
- Li SH, Liao X, Zhou TE, Xiao LL, Chen YW, et al. (2017) Evaluation of 2 Purification Methods for Isolation of Human Adipose-Derived Stem Cells Based on Red Blood Cell Lysis With Ammonium Chloride and Hypotonic Sodium Chloride Solution. Ann Plast Surg. 78(1):83-90.
- Miana VV, Elio APG. (2018) “Adipose tissue stem cells in regenerative medicine.” E Canc Med Sci. 12:822.
- Bartosh, Thomas J, Joni H Ylostalo. (2019) “Efficacy of 3D Culture Priming is Maintained in Human Mesenchymal Stem Cells after Extensive Expansion of the Cells.” Cells. 8(9)1031.
- Klingemann H, Matzilevich D, Marchand J. (2008) Mesenchymal Stem Cells- Sources and Clinical Applications. Transfusion medicine and hemotherapy: offizielles Organ der DeutschenGesellschaft fur Transfusionsmedizin und immunhamatologie. 35(4):272-77.
- Choi EW, Shin IS, Song JW, Lee M, Yun TW, et al. (2016) Effects of Transplantation of CTLA4Ig-Overexpressing Adipose Tissue-Derived Mesenchymal Stem Cells in Mice with Sustained Severe Rheumatoid Arthritis. Cell Transplant. 243-59.
- Liu L, Wong CW, Han M, Farhoodi HP, Liu G, et al. (2019) Meta-analysis of preclinical studies of mesenchymal stromal cells to treat rheumatoid arthritis. E Bio Med. 47:563-77.
- Hassan WU, Greiser U, Wang W. (2014) Role of adipose-derived stem cells in wound healing. Wound Repair Regen. 22(3):313-25.
- Yi T, Song SU. (2012) Immunomodulatory properties of mesenchymal stem cells and their therapeutic applications. Arch Pharm Res. 35(2):213-21.
- Starzl TE, Clyde B. (2009) “The origin of clinical organ transplantation revisited.” JAMA. 301(19):2041-3.
- Till, JE, McCulloch EA. (1961) A Direct Measurement of the Radiation Sensitivity of Normal Mouse Bone Marrow Cells. Radiat Res. 14:213-22.
- Friedenstein AJ, Gorskaja UF, Kulagina NN. (1976) Fibroblast precursors in normal and irradiated mouse hematopoietic organs. ExpHematol. 4:267-74.
- Arutyunyan I, Elchaninov A, Makarov A, Fatkhudinov T. (2016) Umbilical Cord as Prospective Source for Mesenchymal Stem Cell-Based Therapy. Stem cells Int. 6901286.
- Stefańska K, Ozegowska K, Hutchings G, Popis M, Moncrieff L, et al. (2020) “Human Wharton's Jelly-Cellular Specificity, Stemness Potency, Animal Models, and Current Application in Human Clinical Trials.” J Clin Med. 9(4):1102.
- Peberdy L, Young J, Massey D, Kearney L. (2018) “Parents' knowledge, awareness and attitudes of cord blood donation and banking options: an integrative review.” BMC pregnancy and childbirth. 18(1)395.
- Park M, Lee S, Moon S, Lee J, Lee E, et al. (2016) Overexpression of soluble RAGE in mesenchymal stem cells enhances their immunoregulatory potential for cellular therapy in autoimmune arthritis. Sci Rep. 6:35933.
- Zhou Y, Tsai T, Li W. (2017) “Strategies to retain properties of bone marrow-derived mesenchymal stem cells ex vivo.” Annals of the New York Academy of Sciences. 1409(1):3-17.
- Lee R, Kim B, Choi I, Kim H, Choi H, et al. (2004) Characterization and expression analysis of mesenchymal stem cells from human bone marrow and adipose tissue. Cell PhysiolBiochem. 14(4-6):311-24.
- Stanko P, Kaiserova K, Altanerova V, Altaner C. (2014) Comparison of human mesenchymal stem cells derived from dental pulp, bone marrow, adipose tissue, and umbilical cord tissue by gene expression. Biomed Pap Med FacUnivPalacky Olomouc Czech Repub. 158(3):373-77.
- González MA, Gonzalez-Rey E, Rico L, Büscher D, Delgado M. (2009) Treatment of experimental arthritis by inducing immune tolerance with human adipose-derived mesenchymal stem cells. Arthritis Rheum. 60(4):1006-19.
- Mareschi K, Castiglia S, Sanavio F, Rustichelli D, Muraro M, et al. (2015) Immunoregulatory Effects on T lymphocytes by Human Mesenchymal Stromal Cells Isolated from Bone Marrow, Amniotic Fluid and Placenta. ExpHematol. 10:1016.
- Li X, Xu Z, Bai J, Yang S, Zhao S, et al. (2016) Umbilical Cord Tissue-Derived Mesenchymal Stem Cells Induce T Lymphocyte Apoptosis and Cell Cycle Arrest by Expression of Indoleamine 2,3-Dioxygenase. Stem Cells Int. 2016:7495135.
- Zhang Q, Li Q, Zhu J, Guo H, Zhai Q, et al. (2019) Comparison of therapeutic effects of different mesenchymal stem cells on rheumatoid arthritis in mice. PeerJ7:e7023.
- Bourin P, Gadelorge M, Peyrafitte JA, Fleury-Cappellesso S, Gomez M, et al. (2008) Mesenchymal Progenitor Cells: Tissue Origin, Isolation and Culture. Transfus Med Hemother. 35(3):160-7.
- Álvarez-Viejo M. (2020) Mesenchymal stem cells from different sources and their derived exosomes: A pre-clinical perspective. World J Stem Cells. 12(2):100-09.
- Zhang X, Yamaoka K, Sonomoto K, Kaneko H, SatakeM, et al. (2014) Local Delivery of Mesenchymal Stem Cells with Poly-Lactic-CoGlycolic Acid Nano-Fiber Scaffold Suppress Arthritis in Rats. PLoS ONE. 9(12):e114621.
- Raphael I, Joern R, Forsthuber T. (2020) “Memory CD4+ T Cells in Immunity and Autoimmune Diseases.” Cells. 9(3)531.
- Munir S, Basu A, Maity P, Krug L, Haas P, et al. (2020) “TLR4-dependent shaping of the wound site by MSCs accelerates wound healing.” EMBO reports. 21(5):e48777.
- Fabre H, Ducret M, Degoul O, Rodriguez J, Perrier-Groult E, et al. (2019) “Characterization of Different Sources of Human MSCs Expanded in Serum-Free Conditions with Quantification of Chondrogenic Induction in 3D.” Stem cells Int. 2186728.
- Quaedackers ME, Baan CC, Weimar W, Hoogduijn MJ. (2009) Cell contact interaction between adipose‐derived stromal cells and allo‐activated T lymphocytes. Eur J Immunol. 39:3436-46.
- Chinnadurai R, Rajan D, Ng S, McCullough K, Arafat D, et al. (2017) “Immune dysfunctionality of replicative senescent mesenchymal stromal cells is corrected by IFNγ priming.” Blood Adv. 1(11):628-43.
- Levy O, Zhao W, Mortensen L, Leblanc S, Tsang K, et al. (2013) “mRNA-engineered mesenchymal stem cells for targeted delivery of interleukin-10 to sites of inflammation.” Blood. 122(14):e23-32.
- Brand DD, Latham KA, and Rosloniec EF. (2007) Collagen-induced arthritis. Nat Protoc. 2(5):1269-75.
- Schinnerling K, Rosas C, Soto L, Thomas R, Aguillón JC. (2019) Humanized Mouse Models of Rheumatoid Arthritis for Studies on Immunopathogenesis and Preclinical Testing of Cell-Based Therapies. Front Immunol. 10:203.
- Abdelmawgoud H, Saleh A. (2018) Anti-inflammatory and antioxidant effects of mesenchymal and hematopoietic stem cells in a rheumatoid arthritis rat model. AdvClinExp Med. 27(7):873-80.
- Benson R, McInnes I, Garside P, Brewer J. (2018) “Model answers: Rational application of murine models in arthritis research.” Europ J Immunol. 48(1):32-8.
- Yao G, Lam K, Weisemann J, Peng L, Krez N, et al. (2017) “A camelid single-domain antibody neutralizes botulinum neurotoxin A by blocking host receptor binding.” Scientific Rep. 7(1)7438.
- Zhao C, Zhang L, Kong W, Liang J, Xu X, et al. (2015) “Umbilical Cord-Derived Mesenchymal Stem Cells Inhibit Cadherin-11 Expression by Fibroblast-Like Synoviocytes in Rheumatoid Arthritis.” J Immunol Res. 2015:137695.
- Hernandez G, Mills T, Rabe J, Chavez J, Kuldanek S, et al. (2020) “Pro-inflammatory cytokine blockade attenuates myeloid expansion in a murine model of rheumatoid arthritis.” Haematol. 105(3):585-97.
- https://clinicaltrials.gov/ct2/show/NCT01851070
- Monsel A, Zhu Y, Gennai S, Hao Q, Liu J, et al. (2014) “Cell-based therapy for acute organ injury: preclinical evidence and ongoing clinical trials using mesenchymal stem cells.” Anesthesiol. 121(5):1099-121.
- Park KH, Mun CH, Kang MI, Lee SW, Lee SK, et al. (2016). Treatment of Collagen-Induced Arthritis Using Immune Modulatory Properties of Human Mesenchymal Stem Cells. Cell Transplant. 1057-72.
- Park E, Lim H, Lee S, Roh K, Seo K, et al. (2018) Intravenous Infusion of Umbilical Cord Blood-Derived Mesenchymal Stem Cells in Rheumatoid Arthritis: A Phase Ia Clinical Trial. Stem Cells Transl Med. 7(9):636-42.
- Wang L, Huang S, Li S, Li M, Shi J, et al. (2019) Efficacy and Safety of Umbilical Cord Mesenchymal Stem Cell Therapy for Rheumatoid Arthritis Patients: A Prospective Phase I/II Study. Drug Des Devel Ther. 13:4331-40.