Evaluation of the Role of Stem Cells in Treatments for Organ Damage Related to COVID-19
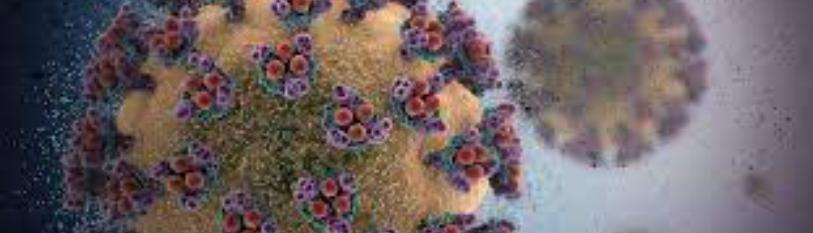
Emma Bryant and Vincent S. Gallicchio*
Department of Biological Sciences, College of Science, Clemson University, Clemson, SC 29636
*Corresponding author: Vincent S. Gallicchio, Department of Biological Sciences, College of Science, Clemson University, Clemson, SC 29636
Citation: Bryant E, Gallicchio VS. (2021) Evaluation of the Role of Stem Cells in Treatments for Organ Damage Related to COVID-19. J Stem Cell Res. 2(2):1-10.
Received: July 14, 2021 | Published: July 30, 2021
Copyright© 2021 genesis pub by Bryant E, et al. CC BY-NC-ND 4.0 DEED. This is an open-access article distributed under the terms of the Creative Commons Attribution-Non Commercial-No Derivatives 4.0 International License., This allows others distribute, remix, tweak, and build upon the work, even commercially, as long as they credit the authors for the original creation.
DOI: https://doi.org/10.52793/JSCR.2021.2(2)-22
Abstract
COVID-19 is an illness that can produce life-threatening complications in hosts. It is caused by the SARS-CoV-2 coronavirus. This work examines and compares existing publications covering the usage of stem cells in the research and treatment of COVID-19 symptoms. Specifically, hPSCs serve a purpose centered on research as they are incorporated into determining pathophysiology of COVID-19; whereas MSC injections are being developed as medical treatments. hPSCs have been utilized to form artificial organoids crucial to the study of COVID-19 effects on the cardiovascular, pulmonary, renal, gastrointestinal, and neural systems. The ability of MSCs to regenerate tissue and communicate with the host immune system to induce anti-inflammatory effects makes them key in medical studies during which MSC solutions are injected into COVID-19-infected patients. The results of these studies, though incomplete, show that MSCs are capable of alleviating COVID-19 symptoms. Both hPSCs and MSCs are therefore suggested to be effective at their particular functions in research. However, hPSC studies pose ethical considerations while those involving MSCs face smaller sample sizes, both of which could impact results. Ultimately, hPSC and MSC studies are limited in their scope due to the novelty of COVID-19, but both are expected to maintain a pivotal role in combating the virus.
Keywords
Mesenchymal stem cells; Induced pluripotent stem cells; Embryonic stem cells; Umbilical cord; Mesenchymal stem cells; COVID-19
Abbreviations
COVID-19: the novel coronavirus; Coronavirus Disease 2019; ACE-2: angiotensin-converting enzyme-2; R0: reproductive number of a communicable pathogen; ARD/ARDS: Acute Respiratory Distress/Acute Respiratory Distress Syndrome; hPSCs: Human pluripotent stem cells; iPSCs: Induced pluripotent stem cells; ESCs: Embryonic stem cells; MSCs: Mesenchymal stem cells; ALI: Air-liquid interface; IDDM: Insulin-dependent diabetes mellitus; UC-MSCs: Umbilical-cord mesenchymal stem cells; BMI: Body mass index
Introduction
Coronavirus Disease 2019, known simply as COVID-19, is a disease caused by the newly emerged virus SARS-CoV-2. This new strain of coronavirus is the third to achieve zoonotic transmission from animal reservoir to humans since the early 2000s, its predecessors being SARS-CoV, first identified in 2003, and MERS-CoV, seen initially in 2013. Originally, COVID-19 was reported in Wuhan, China, in December of 2019 [1]. The exact species of animal reservoir from which the virus spread is unknown, although a genomic sequence identity of 96% was discovered between SARS-CoV-2 and a virus in bats, Bat-CoV-RaTG13, suggesting that SARS-CoV-2 might possess an origin rooted in bats [2].
All coronaviruses are named as such due to the characteristic crown-like spines on their surfaces. These are (S) proteins. Those on the surface of SARS-CoV-2 harbor receptor binding domains (RBDs) fit precisely for the angiotensin-converting enzyme-2 (ACE-2) target molecule in humans. This structural mechanism is inherent to eventual infection of humans since it encourages membrane fusion and ultimate uptake by host cells through endocytosis [2]. Infection is most commonly accomplished when the SARS-CoV-2 virus is transmitted to nearby persons via respiratory aerosol droplets. The pathogen can then interact with host cells. Notably, the (S) proteins in SARS-CoV-2 are of differing structure from those of SARS-CoV and MERS-CoV. This difference could be the driving factor behind the high basic reproductive number (R0) of SARS-CoV-2, which ranges between 2 and 3 people and is larger than both SARS-CoV and MERS-CoV. This means that an individual with COVID-19 infection could be capable of spreading the disease to three additional people on average. Infection by SARS-CoV-2 poses the lowest risk of mortality out of the three at approximately 2% [3]. Despite this, there is a staggering number of COVID-19 cases; as a result, deaths are also in large counts. According to the Centers for Disease Control and Prevention’s COVID Data Tracker, there have been over 27 million cases and about half a million deaths from COVID-19 from its onset to February 2021 [4].
In conjunction with other coronaviruses, SARS-CoV-2 mainly affects the respiratory system. However, a list of COVID-19 symptoms includes, but is not limited to: cough, dyspnea, fever, sore throat, chills, fatigue, headache, loss of smell and taste, nausea, diarrhea, and vomiting. Often, COVID-19 progresses in a three-step pathway, although some recover before experiencing all three levels: Early Infection, Pulmonary Phase, and Hyperinflammatory Phase [2]. The first phase is characterized by mild flu-like symptoms. The second poses a significant handicap to the lungs. Pneumonia, dyspnea, and presence of lung opacities on chest radiographs as a result of lowered oxygen availability are hallmarks of this stage. The third phase leads to organ failure, Acute Respiratory Distress (ARD), sepsis, and sometimes death. Also, during this stage, a cytokine storm phenomenon takes place, in which cytokines are produced in excess by the body in an effort to thwart the virus yet are unfortunately often responsible for serious hyperinflammation, which can direct the progression of organ failure [2].
Treatment of COVID-19 is still controversial. Although a specific treatment for COVID-19 is not yet available, a multitude of drugs have been used in an effort to alleviate or prevent symptoms. Some of these include azithromycin, hydroxychloroquine, corticosteroids, vitamin C, vitamin D, and zinc [2]. However, most require a combination of other drugs and are not scientifically determined to be effective through sufficient conduction of clinical trials. This can lead to adverse effects or side effects, as well as dangerous drug interactions. Another treatment involving stem cells is in its infancy and may be a natural strategy, engineered by human bodies themselves, to significantly reduce COVID-19 effects such as organ damage resulting from the cytokine storm.
An Exploration of Stem Cells
Stem cells are unspecialized cells generated by the body that have the power both to regenerate additional stem cells and to transform into any type of specialized cell through differentiation. Cells that are already specialized cannot convert daughter cells into a differing type. Although the role of stem cells is most obvious during the embryonic stage, they remain significant throughout the entire lifespan, granting the body its ability to regenerate itself following injury and replace aged or defective cells. Adult stem cells can be utilized to perform functions of the various cells in the bloodstream, bones, skin, and nervous system, for example. In medical settings, stem cells are already being utilized to replace cells damaged by chemotherapy through bone marrow transplants. Research into other uses for stem cells in medicine is being conducted, especially in the wake of the COVID-19 pandemic.
Totipotent stem cells and pluripotent embryonic stem cells possess the most extensive differentiation abilities but can also be the most controversial. Totipotent stem cells are those capable of transforming into any cell of the body and out of it, including placental cells. The best example of this category is a single-celled human zygote. In comparison, pluripotent stem cells cannot form the placenta as totipotent cells can, but they are still able to transform into any cell inside the body. The most abundant source of pluripotent stem cells is the multicellular human embryo [5]. Treatments involving cells of either the totipotent or pluripotent type are often regarded as controversial as they often require the termination of cells which have the potential to form live human offspring. There is no published evidence suggesting that any research dealing with COVID-19 has ever recruited totipotent zygotes. The focus of many studies and publications has been on human pluripotent stem cells (hPSCs), under which induced pluripotent (iPSCs) and embryonic (ESCs) cells can be categorized, and multipotent cells.
In contrast with general pluripotent cells, hPSCs and multipotent cells do not rely on antepartum elements as a main source of stem cells. Instead, hPSCs contain iPSCs on top of ESCs. iPSCs are developed from somatic cells whose genomes have been artificially amended to contain sections of DNA responsible for maintaining embryonic properties. This specific genetic information is typically lost by the embryo as it develops. As a result of this alteration, iPSCs are able to differentiate almost as extensively as natural pluripotent cells. Genetic engineering may be costly and timely; however, using iPSCs avoids potential backlash that might have resulted from utilization of zygotic or embryonic stem cells. Lastly, multipotent cells have the most limited ability to differentiate, only capable of developing as the cells in a certain bodily region, system, or organ. For example, hematopoietic stem cells can mature into various types of cells found in the bloodstream [5]. Despite restricted capabilities, multipotent cells might be easier to experimentally control than cells with more complex abilities. Mesenchymal stem cells (MSCs) are multipotent and are crucial to current COVID-19 medical treatments. Ultimately, both hPSCs and MSCs will serve as the principal focus in this review as the following question is explored: To what extent can the abilities of stem cells be harnessed in order to alleviate organ damage as a result of COVID-19 infection?
Studies outlining the methods by which the effect of COVID-19 on human organs can be ethically observed through use of pluripotent stem cells, as well as those studies surrounding the role of MSCs, both in tissue repair of cell clusters already damaged by COVID-19 viral infection and in overall prevention of organ injury through effective treatment of COVID-19, reveal a promising future for stem cells in virology.
Discussion
The hPSC as a way to study covid-19 effects
Scientific research is always accompanied by strong ethical considerations. The quest for increased medical understanding, for this reason, is no easy challenge. Many medical studies on human conditions and disease require recruitment of human participants. A large majority of surveyors cannot guarantee to subjects that they will be fully informed of all possible health risks the experiment may pose. This then also means that the “do no harm” principle of medical ethics might not be upheld by evaluators, many of whom are healthcare professionals, which can disrupt the imperative bond of trust between patient and medical provider. Medical researchers and potential civilian participants can find experimentation understandably daunting as a result. While raising ethical concerns in medical research has likely protected some from suffering adverse or potentially disastrous effects of experimentation, it has limited the scope of medical knowledge. This can restrict the development of life-saving medical advances. As a much safer alternative, researchers have begun to utilize iPSCs harvested from adult somatic cells as subjects in order to further accurate medical research in those cases where using humans is unethical, risky, or otherwise impossible. iPSCs can be artificially directed through genetic engineering to transform into an organoid of interest that is ideal for experimentation and testing. In this way, iPSCs can grant medical researchers a glimpse into the workings of the body and the manner in which it is affected by external factors, all without posing significant risk to human beings.
Frequently in the case of COVID-19, iPSCs are engineered to form infected organoids in order to isolate the effects of the virus on particular bodily structures. For example, a 2020 study by Loukia Yiangou and colleagues investigates the incorporation of hPSCs into the study of COVID-19 infection effects on the cardiovascular system. The publication reports that 20-30% of all those infected with COVID-19 will face severe cardiac damage in a variety of forms, including arrhythmias, acute coronary syndrome, and both vascular and myocardial injury. Thus, the study of the relationship between the novel coronavirus and the heart as a vital organ has been prioritized. It is suggested that hPSCs can be used to derive models mimicking conditions of atherosclerosis, ischemia-related damage, fibrosis, and hypertension in the heart, which can then be infected with SARS-CoV-2 in order to determine the impact of infection on pre-existing conditions. hPSCs can also be used to determine risk of COVID-19 severity for a variety of groups, including those categorized by sex, race, and socioeconomic status, through introduction of appropriate genetic variations to hPSCs. The organoids can also be exposed to pharmaceuticals prior to and after infection. This can be helpful in determining potential remedies for COVID-19 symptoms and possibly a cure for the sickness itself. They can also be used to highlight infection routes taken by the virus within the body as alternatives from the originally hypothesized ACE2 pathway. Moreover, hPSC-derived organoids have already revealed that damage to the endothelium of the hPSCs can occur post-exposure despite an apparent absence of successful infection by SARS-CoV-2. Additional effects on both evidently infected and uninfected hPSCs after viral exposure include: contractile dysfunction, apoptosis, loss of nuclear DNA, sarcomeric fragmentation, and arrhythmias due to, but not limited to, diminished depolarization spike amplitude and reduced electrical conduction velocity in electrical impulses of the heart. Finally, hPSCs are predicted to be instrumental in gathering scientific data on long-term effects of COVID-19 [6].
The main advantage of this article is that it proposes a plethora of strategies for use of stem cells. It also describes the steps for each procedure in comprehensive detail, and the facts are presented in a logical sequence. However, it is important to note that a majority of the suggestions for stem cell use in the publication are hypothetical and are not attached to any facts. It is unknown if stem cell research would best answer some of the unanswered questions presented. Such queries are of high number given the novelty of the 2019 coronavirus pandemic, and much of the research findings in the article require supplemental supporting information. They are therefore subject to change. Exact causes for many of the cardiovascular conditions expressed cannot be pinpointed as the research is still in early infancy; it is equally likely that cellular damage could be the result of immune response as opposed to direct infection.
Additionally, hPSCs are utilized in research of pulmonary, renal, gastrointestinal, and neural systems. A 2021 article authored by Rishi Man Chugh and colleagues describes various stem cell-derived models for research. This publication identifies an air-liquid interface (ALI) structure, in which iPSCs divide to maturity and fill an unattainable filter with access to air, as the best artificial recreation of a human lung. However, because they do not possess stroma and immune cells, the pseudo-lungs can also be illustrated by an alternative prototype known as the “lung-on-a-chip” model. These lungs, formed by hPSCs, are connected to channels participating into a lifelike circulatory process that delivers immune cells to the artificial structures for results more representative of genuine human lungs. Data is collected through a microchip embedded in the model. Advances created with both structures were able to determine three SARS-CoV-2 entry inhibitors in the pulmonary system: imatinib, mycophenolic acid, and quinacrine dihydrochloride. Stem cell-derived organoids have also been used to investigate COVID-19 effects on renal, gastrointestinal, and neural systems. Kidney models have revealed that combining the use of antiviral Remdesivir with the human recombinant soluble ACE-2 can effectively minimize infection by COVID-19 pathogens. Gastrointestinal models show that gut enterocytes are highly susceptible to COVID-19 infection due to their enrichment in and expression of ACE-2 and serine proteases, which can alter the virus in a way that promotes its entry into the body. Finally, artificial models of the brain indicate that infection leads to metabolic changes within neurons, and the brain is uniquely vulnerable in that infection tends to alter pathways crucial to cell division in this area [7].
A strong benefit to the publication by Man Chugh’s team is that it portrays a clear impartiality to stem cell research and the other topics it discusses. Great detail is placed on that which has already been accomplished by scientific developments like artificial organoids, but limitations are still explicitly stated and explained, nevertheless. However, the work does have a broad scope, and as a result, much of the discussion in it is short and slightly vague compared to the publication by Yiangou’s team, which focuses on one body system instead of multiple. Similar to Yiangou’s team, though, is the inevitable incompletion of most study results cited, merely due to the novelty of the coronavirus pandemic. The virus has only recently been identified and it is certain that more experimentation should be conducted in order to either challenge or support pre-existing findings.
The Role of MSCs in Repair of Tissue Damaged by COVID-19 Infection
Whereas hPSCs appear to be used most widely in COVID-19 medical research, multipotent cells such as MSCs are vital in the treatment of the virus and mitigation of bodily damage that commonly results in hospitalized patients. This is likely due to the fact that MSCs are readily available in healthy adults and are relatively easy to harvest from donors. They also have strong differentiation abilities. Unlike iPSCs, genetic engineering is not required to grant MSCs status as stem cells, given that the correct type of MSCs have been collected. The transfer process from donor to recipient is largely straightforward for MSCs but is more complex for hPSCs. MSCs are also significantly less controversial than hPSCs because they do not involve antepartum products in any capacity. Apart from these reasons, three specific capabilities of MSCs have allowed them to take center stage in the development of COVID-19 treatment options:
Minimal adverse effects of treatment
One of the main risks of note in regenerative medicine is the possibility of tumorigenesis within a patient sometime after treatment. Concern arises from the biology of MSCs, specifically in that they are highly viable and resistant to cellular mechanisms for apoptosis. However, MSCs also have anti-tumorigenic capabilities since they can minimize migratory tendencies of malignant cells, as stated in a publication by Aleksandra Musial-Wysocka’s team [8]. Nevertheless, should they be performed, many studies suggest that treatments using MSCs are not extensively dangerous. One reports that out of 34 patients in a study lasting over two years, none reported serious adverse reactions to MSC treatment, and MRI results for each were all unremarkable. Additional research is required to fully examine the long-term effects of this treatment. Other risks of MSCs exist, such as pulmonary embolism as a result of MSC coagulatory tendencies, but more research is needed to determine the extent to which this is a true possibility with MSC therapy [8].
Interaction with the immune system bolsters MSC anti-inflammatory abilities:
Communication between the immune system of the host and introduced MSCs enables MSCs, specifically, to possess immunomodulatory properties. MSCs are crucial in subduing the cytokine storm present in many COVID-19-infected patients through their release of multiple chemical factors in various combinations that enable MSCs to influence several bodily mechanisms. They are able to inhibit T-cell activation that occurs prior to the storm and can also increase concentrations of anti-inflammatory TH2 cells as well as regulatory T-cells. At the same time, MSCs can increase anti-inflammatory M2 macrophage polarization. Combined, these capabilities enable MSCs to significantly reduce the effects of the cytokine storm, prevent resulting organ damage, and reduce the mortality rate of COVID-19 [9].
Tissue regeneration:
Interplay between MSCs and the host immune system can not only promote anti-inflammatory responses but can also encourage the healing process. MSC secreting factors can facilitate growth of additional cells, promote neovascularization, reduce scarring, and in lungs, promote alveolar epithelial cell regeneration, which would be particularly useful in the treatment of COVID-19-infected patients MSCs are typically anti-apoptotic and therefore can be used to curb effects of organ failure. Inserted after a myocardial infarction, MSCs can be useful in preventing accumulation of potentially harmful scar tissue, and injection close to onset of insulin-dependent diabetes mellitus (IDDM) has been shown to prevent autoimmune attack in β-islets. This can temporarily restore glucose modulatory capabilities in the body [10]. Ultimately, the methods by which MSCs actually promote tissue repair have not been fully elucidated, and more is still being learned about MSCs and their function.
Given the extensive abilities of MSCs, they have been incorporated into multiple treatments designed to address the many symptoms and complications of COVID-19. One study recruited seven COVID-positive patients for whom conventional treatments had not been effective. Two of the patients were suffering from a moderate or common case, four were classified as possessing a severe case, and one was declared critically severe. Three of the severe cases were assigned a placebo, and the remaining were given one intravenous dose of ACE-2 MSC solution. By the end of the fourth day post-treatment, all experimental patients no longer had high temperatures, shortness of breath, weakness, and low oxygen saturation. In fact, they had above 95% oxygen saturation at rest. None experienced any infection as a result of the procedure. At the conclusion of the 14-day assessment period, all had oxygen saturation levels above 98%, and the critically severe patient experienced a significant improvement of lymphopenia [11]. This study suggests that MSCs are effective in reducing the severity of COVID-19 and COVID-19-related pneumonia. It also is an indicator that MSC transfer could be suitable for regular use in hospitals, and the benefits of such a procedure might outweigh possible risks.
This study reveals promising results for regenerative medicine. The project offers informative medical background on each patient and results of medical testing after the MSC treatment is clearly displayed. Moreover, classifications of “mild”, “common”, “severe”, and “critically severe” are explicitly defined. In an effort to avoid unforeseen effects on results, only patients who had not participated in another clinical trial up to three months prior were eligible. However, the study includes only seven patients, and they were studied for less than a month. The small sample size could have easily been impacted by extraneous variables, which would skew results; additionally, this is not an accurate representation of the entire population of COVID-19 patients. The study also does not reveal much about the long-term effects of MSC treatment, particularly in the case of COVID-19 symptom alleviation. More research will be needed with larger sample sizes over a longer assessment period in order to obtain a more accurate picture of MSC treatment and its impacts.
Furthermore, a separate study reports the effects of umbilical cord MSCs (UC-MSCs) on the severity of ARDS in COVID-19 patients. It has been described as a double-blind control trial, in which a total of 24 COVID-19-infected subjects were randomly assigned in a 1:1 ratio to either the placebo or experimental group. The latter was given a solution on two separate occasions containing MSCs collected from umbilical cords separated from recently delivered infants. Three in each group were classified as mild-to-moderate, while the remaining participants were deemed moderate-to-severe. The rate of comorbidities, especially between body mass index (BMI) and obesity, was higher in the UC-MSC group. Results included a total of nine deaths after the 28th day and the second infusion, seven of those from the control group and one of the two in the UC-MSCs resulting from a failure to successfully intubate instead of directly from COVID-19 infection. Moreover, two serious adverse effects were noted in the experimental group, while 16 were observed in the control group. Patient survival in the experimental group was 91% as opposed to 42% in the control group, and there was a significant decrease in recovery time in the UC-MSC group. Ultimately, the publication concludes that MSC treatment is safe and may pose many beneficial effects for those infected with COVID-19, including lower risk of mortality, decreased severity of symptoms, and less recovery time [12]. This publication is yet another of several studies to encourage the therapeutic use of MSCs. There is a clear difference in prognosis between the experimental and control groups.
Similar to the previous study on ACE-2 MSCs, the publication on UC-MSCs presents positive results overall. Both include clear medical backgrounds on their patients, and potential participants were screened for eligibility prior to their official enrollment into the studies. However, the review of UC-MSCs might be slightly more representative of the actual population of COVID-19-infected patients because of its larger sample size. This study also emphasized its status as double-blind, which could eliminate much of the bias that could have otherwise been introduced by both patients and healthcare officials. Despite this, the UC-MSC study only lasted slightly longer than the ACE-2 MSC one, that is, for three months as opposed to just one. As already mentioned, additional research is needed to shed more light on the effectiveness, or lack thereof, of MSC treatment of COVID-19, and the potential long-term impacts it may present.
Conclusion
It is strongly evident that stem cells are key elements of medical research into COVID-19, with hPSCs more involved in determining pathophysiology of the virus and MSCs utilized as treatment mechanisms. hPSCs have been critical to pinpointing the areas and systems of the body most dramatically affected by COVID-19; therefore, they serve as the foundation for many medicaments as well as possible vaccines developed to combat the novel coronavirus. However, they pose a special challenge in that some consider them ethically unsound due to the incorporation of embryonic cells into hPSC mixtures. MSCs do not directly involve any antepartum elements but can be limited in their ability to differentiate into desired cells. The donor must possess sufficient levels of the specific type of MSC required and once collected, they can be difficult and time-consuming to separate from other cell types. hPSCs can be used for a wider variety of procedures and treatment plans. Moreover, all studies require an adequate sample size, which is a conflict that is relatively easy to solve for those involving hPSCs since they rely on artificially created organoids for experimental subjects. Most of the hPSC studies focus on the effect of COVID-19 on the particular body part that the organoid symbolizes. On the other hand, MSC treatments typically demand studies recruiting live humans since MSCs are being tested more as injectable remedies for the virus and, as a result, must be tested for their effectiveness at alleviating viral symptoms and their holistic impacts on the body. Humans as experimental subjects are difficult to obtain in satisfactory numbers because risk cannot be completely calculated. Smaller sample size can significantly influence the collected results, which could have possibly occurred in previously discussed studies involving MSCs. Despite these differences, usage of hPSCs and MSCs is united by uncertainty. Gaps exist in the data due to small sample size, short observation period, and unforeseen circumstances such as sudden patient death before conclusion of the study. These are inevitable given the novelty of COVID-19. Medical and scientific knowledge on the virus is in its infancy. As time bears on, participant hesitancy will wane as comprehension of the illness grows, and it will be possible to determine the long-term effects both of COVID-19 and of stem cell use. Currently, however, conducted studies agree that stem cells are beneficial to research and suggest that MSCs are effective at moderating even the most severe of COVID-19 symptoms.
References
- https://www.cdc.gov/coronavirus/2019-ncov/cdcresponse/about-COVID-19.html
- Dos Santos WG. (2020) Natural history of COVID-19 and current knowledge on treatment therapeutic options. Biomed Pharmacother. 129:110493.
- Petrosill N, Viceconte G, Ergonul O, Ippolito G, Petersen E. (2020) COVID-19, SARS and MERS: Are they closely related? Clin Microbiol Infect. 26(6):729-34.
- https://covid.cdc.gov/covid-data-tracker/#cases_casesper100klast7days
- Zakrzewski W, Dobrzyński M, Szymonowic M, Rybak Z. (2019) Stem cells: past, present, and future. Stem cell res & therap. 10(1):68.
- Yiangou L, Davis RP, Mummery CL. (2021) Using Cardiovascular Cells from Human Pluripotent Stem Cells for COVID-19 Research: Why the Heart Fails. Stem cell reports. 16(3):385-97.
- Chugh RM, Bhanja P, Norris A, Saha S. (2021) Experimental Models to Study COVID-19 Effect in Stem Cells. Cells. 10(1):91.
- Musiał-Wysocka A, Kot M, Majka M. (2019) The Pros and Cons of Mesenchymal Stem Cell-Based Therapies. Cell transplant. 28(7):801-12.
- Sadeghi S, Soudi S, Shafiee A, Hashemi SM. (2020) Mesenchymal stem cell therapies for COVID-19: Current status and mechanism of action. Life sciences. 262:118493.
- Parekkadan B, Milwid JM. (2010) Mesenchymal stem cells as therapeutics. Annu Rev Biomed Eng. 12:87-117.
- Leng Z, Zhu R, Hou W, Feng Y, Yang Y, et al. (2020) Transplantation of ACE2-Mesenchymal Stem Cells Improves the Outcome of Patients with COVID-19 Pneumonia. Aging Dis. 11(2):216-28.
- Lanzoni G, Linetsky E, Correa D. (2021) Umbilical cord mesenchymal stem cells for COVID‐19 acute respiratory distress syndrome: A double‐blind, phase 1/2a, randomized controlled trial. STEM CELLS Transl Med. 2021:1-14.