Biologics that increase Chondrocyte Number and/or Matrix
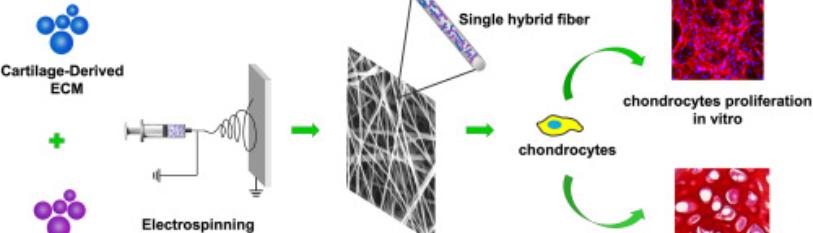
Gordon Slater*, Luke Richardson
Orthopaedic Surgeon, Private Practice, Level 2, Suite 2.01,376 New South Head Rd, Double Bay, NSW 2028; Australia
*Corresponding author: Gordon Slater, Orthopaedic Surgeon, Private Practice, Level 2, Suite 2.01,376 New South Head Rd, Double Bay, NSW 2028; Australia
Citation: Slater G, Richardson L. (2021) Biologics that increase Chondrocyte Number and/or Matrix. J Stem Cell Res. 2(2):1-5.
Received: April 15, 2021 | Published: April 30, 2021
Copyright© 2021 genesis pub by Slater G, et al. CC BY-NC-ND 4.0 DEED. This is an open-access article distributed under the terms of the Creative Commons Attribution-Non Commercial-No Derivatives 4.0 International License., This allows others distribute, remix, tweak, and build upon the work, even commercially, as long as they credit the authors for the original creation.
DOI: https://doi.org/10.52793/JSCR.2021.2(2)-20
Abstract
Cartilage injuries and disease are observed in a varied range of people; for instance, cartilage defects in juveniles, osteoporosis and osteoarthritis in the aged population. Cartilage injuries frequently result in disability and a probable demand for invasive surgery in future. Chondrocytes proliferate and synthesize extracellular matrix in order to maintain the cartilage. These cells are separated from each other by cartilage matrix. The aim of the present study was to understand the roles of the biologics in in cartilage repair, cartilage regrowth and regeneration, chondrocytes proliferation plus differentiation, and matrix production. It is also highlighted the necessity of further investigations into the biologics to regrow cartilage by in vitro studies and animal models.
Keywords
Cartilage; Regeneration; Chondrocyte; Matrix; Cartilage repair; Cartilage defect; Slater
Introduction
Cartilage is essential for the healthy function of the human skeleton. In the skeletal system, cartilage provides a means for bones to grow in the young, for the articulation of joints, shock absorption and maintenance the structure of the skeleton [1]. Despite its important role in the skeleton, cartilage has a limited ability to heal and regenerate [2,1]. Damaged/lost cartilage can be caused by trauma [3] and pathologies of the musculoskeletal system including osteoarthritis (OA) [4], endocrine pathologies [5], cancer [6] and congenital diseases [7]. Because the body has a limited ability to heal damaged cartilage on its own [2,1], it is of interest to develop methods by which cartilage can be regenerated. This article reviews some of the biologics (including cells, matrix scaffolds and proteins) that have had success in increasing the number of chondrocytes, the amount of cartilaginous matrix, or both.
Body
One of the first instances of biologics being utilised in cartilage repair is that of autologous chondrocyte implantation (ACI), where chondrocytes are extracted from a patient and grown ex vivo, before being re-implanted into the patient [8]. The technique was successful in regrowing articular cartilage of the knee in patients with deep cartilage defects [8]. Since then, this technique has been further developed into matrix-associated autologous chondrocyte implantation (MACI), where healthy cells are harvested from a patient, seeded into a collagen matrix and then re-implanted into a patient’s cartilage defect [9]. Another technique is that of autologous matrix-induced chondrogenesis (AMIC), a technique in which a cell-free collagen type I/III matrix is implanted into a defect, usually of the knee or ankle [10]. More recently, micro fracture in combination with delivery of BMP2 and soluble VEGFR1 has been shown to promote skeletal stem cell (SSC) differentiation into chondrocytes on chondral surfaces of adult mice [11].
Growth factors and other proteins have also been investigated for their ability to re-grow cartilage. In transfected rat chondrosarcoma cells and human primary chondrocytes, granulin-epithelin precursor protein (GEP) has been shown to induce proliferation of chondrocytes, which is potentiated by human cartilage oligomeric matrix protein (COMP) [12]. In a mouse model of OA, a helper-dependent adenovirus was used to transfect interleukin-1 receptor antagonist (IL-1a), which increased cartilage volume and surface [13]. Human growth hormone (HGH) is known to induce proliferation of rabbit and rat chondrocytes [14], an effect that is likely mediated largely through the action of insulin-like growth factor 1 (IGF-1) [15]. Injections of hGH into the arthritic temporomandibular joint of rats also improved their OA scores [16], and has improved healing in arthritic knees to a greater extent than hyaluronic acid in a rabbit model of OA [5].
Transforming growth factor-beta1 (TGF-β1) has exhibited the ability to promote rat bone marrow mesenchymal stem cells into chondrocytes via MAPK [17]. Moreover, TGF-β1 promoted production of cartilage matrix in rabbits, even without administering mesenchymal stem cells [18]. Treatment with TGF-β1 also increased production of lubricin, a component of the chondrocyte matrix, by human chondrocytes in vitro [19]. Furthermore, TGF-β1 promoted mesenchymal stem cell differentiation into chondroctyes, and promoted cartilage formation in tandem with SDF-1α [20]. Finally, TGF-β1 and BMP2 have been delivered via nanoparticles used in combination with a silk fibroin hydrogel to repair cartilage defects in rabbits [21]. Not only has the CS (Chitosan) hydrogels loaded with TGF-β1 been proved in supporting chondro-inductive therapies [22], but also the CS-coated SF (silk fibroin) scaffolds in combination with BMP-2 have been demonstrated to stimulate bone defect regeneration [23]. Moreover, possessing remarkable bone formation abilities at the defect area, TGF-β1@CS/BMP-2@SF was shown to promote chondrogenic ability of BMSCs since CS/SF can accelerate chondrocyte-like cell adhesion, matrix production, and proliferation [24]. Clearly, BMSCs expressing TGF-β1 have been seen to cause cartilage defect repair that is composed of a larger number of GAG and collagen II [25], which are increased in BMSCs co-cultured with TGF-β1@CS/BMP-2@SF, suggesting improved cartilage defects [21]. Furthermore, TGF-β1@CS/BMP-2@SF can contribute to formation of cartilage lacuna in cell mass, besides multiplication of proteoglycans in the extracellular matrix. Similarly, an increase in the GAG content by 15% TGF-β1@CS/BMP-2@SF was observed (Figure 1). Fascinatingly, postoperative morphological views in defect areas after a couple of weeks, have exhibited the presence of hyaline cartilage and repair tissues with smooth surface integrated with adjacent cartilage, and a small number of infiltrated inflammatory cells in the TGF-β1@CS/BMP-2@SF treated group [21].
Figure 1: The GAG content increases in the presence of 15% TGF-β1@CS/BMP-2@SF detected by Alcian blue colorimetric method.
Discussion
While usage of cells and/or matrix implantation shows several examples of success in patient outcomes, certain cases may indicate a decreased chance of positive outcomes. For example, although exhibiting success in treating defects of the ankle [9,26] and simple defects of the knee [27], MACI has shown limited success and poor outcomes in complex and salvage cases of the knee, respectively [27]. Further investigation into promotion of SSC differentiation [11] may help discern whether or not such a technique may present a possible alternative in such cases, as it is yet to be trialed in human patients.
The use of proteins in inducing chondrocyte growth and matrix production appears to have promise. TGF-β1 has shown great potential as a biologic for cartilage regeneration in animal models, and in human cell lines, exhibiting an ability to regrow the cartilage matrix even without transfected cells [20]. As well as this, viral vectors, like adenovirus, may be used to administer biologics such as IL-1a, abrogating the ability of cytokines to interfere with chondrocyte differentiation and production of the cartilage matrix [13,28]. hGH was successful in improving the condition of OA in the temporomandibular joint of rats [16], as well as in the knees of rabbits, to a greater extent than hyaluronic acid [29,30]. It is interesting to note that the injection of hGH in the arthritic joint of the rats increased IGF-1 concentrations in the joint, but not in the animal’s serum [16], which could indicate a decreased chance of off-target actions by IGF-1 following HGH injections into the joint. These investigations into proteins to regrow cartilage are largely limited to in vitro studies and animal models, however, and thus warrant further investigation.
Disclosure
Dr. Gordon Slater is medical director of Integrant Pty Ltd an orthobiologics company. He is a former director of Albury Day Surgery.
References
- Sophia Fox AJ, Bedi A, Rodeo SA. (2009) The basic science of articular cartilage: structure, composition, and function. Sports Health. 1(6):461-68.
- Hunter W.Philosophical Transactions (1683-1775), 1742 - 1743, Vol. 42 (1742 - 1743):514-21.
- Torzilli PA, Grigiene R, Borrelli J, Jr Helfet DL. (1999) Effect of impact load on articular cartilage: cell metabolism and viability, and matrix water content. J Biomech Eng. 121(5):433-41.
- Grassel S, Aszodi A.(2019) Osteoarthritis and Cartilage Regeneration: Focus on Pathophysiology and Molecular Mechanisms. Int J Mol Sci. 20(24).
- Liote F, Orcel P. (2000) Osteoarticular disorders of endocrine origin. Baillieres Best Pract Res Clin Rheumatol .14(2):251-76.
- Martel-Pelletier J, Pelletier JP. (1986) Degradative changes in human articular cartilage induced by chemotherapeutic agents. J Rheumatol/ 13(1):164-174.
- Krishnan Y, Grodzinsky AJ. (2018) Cartilage diseases. Matrix Biol. 71-72:51-69.
- Brittberg M, Lindahl A, Nilsson A, Ohlsson C, Isaksson O. (1994) Treatment of deep cartilage defects in the knee with autologous chondrocyte transplantation. N Engl J Med 331(14):889-95.
- Cherubino P, Grassi FA, Bulgheroni P, Ronga M. (2003) Autologous chondrocyte implantation using a bilayer collagen membrane: a preliminary report. J Orthop Surg (Hong Kong). 11(1):10-15.
- Benthien JP, Behrens P. (2010) Autologous Matrix-Induced Chondrogenesis (AMIC): Combining Microfracturing and a Collagen I/III Matrix for Articular Cartilage Resurfacing. Cartilage. 1(1):65-8.
- Murphy MP, Koepke LS, Lopez MT, Tong X, Ambrosi TH, et al. (2020) Articular cartilage regeneration by activated skeletal stem cells. Nat Med. 26(10):1583-92.
- Xu K, Zhang Y, Ilalov K, Carlson CS, Feng JQ, et al. (2007) Cartilage oligomeric matrix protein associates with granulin-epithelin precursor (GEP) and potentiates GEP-stimulated chondrocyte proliferation. J Biol Chem. 282(15):11347-355.
- Nixon AJ, Grol MW, Lang HM, Ruan MZC, Stone A, et al. (2018) Disease-Modifying Osteoarthritis Treatment With Interleukin-1 Receptor antagonist gene therapy in small and large animal models. Arthritis Rheumatol . 70(11):1757-68.
- Madsen K, Friberg U, Roos P, Eden S, Isaksson O. (1983) Growth hormone stimulates the proliferation of cultured chondrocytes from rabbit ear andrat rib growth cartilage. Nature. 304(5926):545-47.
- Tsukazaki T, Matsumoto T, Enomoto H, Usa T, Ohtsuru A,et al. (1994) Growth hormone directly and indirectly stimulates articular chondrocyte cell growth. Osteoarthritis Cartilage. 2(4):259-67.
- Ok SM, Kim JH, Kim JS, Jeong EG, Park YM, et al. (2020) Local Injection of Growth Hormone for Temporomandibular Joint Osteoarthritis. Yonsei Med J. 61(4):331-40.
- Li J, Zhao Z, Liu J, Huang N, Long D, et al. (2010) MEK/ERK and p38 MAPK regulate chondrogenesis of rat bone marrow mesenchymal stem cells through delicate interaction with TGF-beta1/Smads pathway. Cell Prolif. 43(4):333-43.
- Re’em T, Witte F, Willbold E, Ruvinov E, Cohen S. (2012) Simultaneous regeneration of articular cartilage and subchondral bone induced by spatially presented TGF-beta and BMP-4 in a bilayer affinity binding system. Acta Biomater. 8(9):3283-93.
- Iwasa K, Reddi AH. (2017) Optimization of Methods for Articular Cartilage Surface Tissue Engineering: Cell Density and Transforming Growth Factor Beta Are Critical for Self-Assembly and Lubricin Secretion. Tissue Eng Part C Methods. 23(7):389-95.
- Chen Y, Wu T, Huang S, Suen CW, Cheng X, et al. Sustained Release SDF-1alpha/TGF-beta1-Loaded Silk Fibroin-Porous Gelatin Scaffold Promotes Cartilage Repair. ACS Appl Mater Interfaces. 11(16):14608-18.
- Li Y, Liu Y, Guo Q. (2021) Silk fibroin hydrogel scaffolds incorporated with chitosan nanoparticle repair articular cartilage defects by regulating TGF-beta1 and BMP-2. Arthritis Res Ther. 23(1):50.
- Xu Z, Shi L, Yang M, Zhu L. (2019) Preparation and biomedical applications of silk fibroin-nanoparticles composites with enhanced properties - a review. Mater Sci Eng C Mater Biol Appl. 95:302-11.
- Wu J, Zheng A, Liu Y, Jiao D, Zeng D, et al. (2019) Enhanced bone regeneration of the silk fibroin electrospun scaffolds through the modification of the graphene oxide functionalized by BMP-2 peptide. Int J Nanomedicine. 14:733-51.
- Silva SS, Motta A, Rodrigues MT, Pinheiro AF, Gomes ME, et al. Novel genipin-cross-linked chitosan/silk fibroin sponges for cartilage engineering strategies.Biomacromolecules. 9(10):2764-74.
- Ying J, Wang P, Zhang S, Xu T, Zhang L, et al. (2018) Transforming growth factor-beta1 promotes articular cartilage repair through canonical Smad and Hippo pathways in bone mesenchymal stem cells. Life Sci. 192:84-90.
- Ronga M, Grassi FA, Montoli C, Bulgheroni P, Genovese E, et al. (2005) Treatment of deep cartilage defects of the ankle with matrix-induced autologous chondrocyte implantation (MACI). Foot and Ankle Surg. 11(1):29-33.
- Brix MO, Stelzeneder D, Chiari C, Koller U, Nehrer S, et al. (2014) Treatment of Full-Thickness Chondral Defects With Hyalograft C in the Knee: Long-term Results. Am J Sports Med. 42(6):1426-32.
- Wehling N, Palmer GD, Pilapil C, Liu F, Wells JW, et al. (2009) Interleukin-1beta and tumor necrosis factor alpha inhibit chondrogenesis by human mesenchymal stem cells through NF-kappaB-dependent pathways. Arthritis Rheum. 60(3):801-12.
- Lubis AMT, Wonggokusuma E, Marsetio AF. (2019) Intra-articular Recombinant Human Growth Hormone Injection Compared with Hyaluronic Acid and Placebo for an Osteoarthritis Model of New Zealand Rabbits. Knee Surg Relat Res. 31(1):44-53.
- Choi B, Kim S, Fan J, Kowalski T, Petrigliano F, et al. (2015) Covalently conjugated transforming growth factor-beta1 in modular chitosan hydrogels for the effective treatment of articular cartilage defects. Biomater Sci. 3(5):742-52.