In Utero Hematopoietic Stem Cell Transplantation
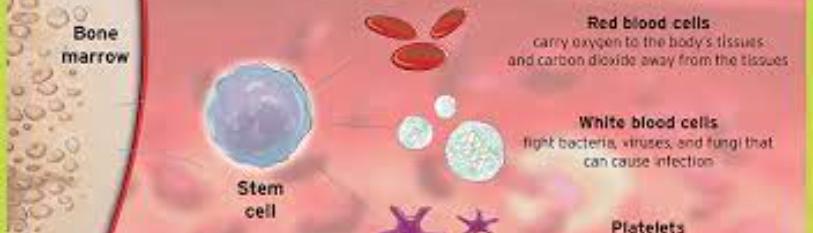
Charlotte M Vogel and Vincent S. Gallicchio*
Department of Biological Sciences, College of Science, Clemson University, Clemson, South Carolina, USA
*Corresponding author: Vincent S Gallicchio, Department of Biological Sciences, College of Science, Clemson University, Clemson, South Carolina, USA
Citation: Charlotte M Vogel, Gallicchio VS. (2021) In Utero Hematopoietic Stem Cell Transplantation. J Stem Cell Res. 2(2):1-15.
Received: June 18, 2021 | Published: July 02, 2021
Copyright© 2021 genesis pub by Vogel CM, et al. CC BY NC-ND 4.0 DEED. This is an open-access article distributed under the terms of the Creative Commons Attribution-NonCommercial-No Derivatives 4.0 International License., This allows others distribute, remix, tweak, and build upon the work, even commercially, as long as they credit the authors for the original creation.
DOI: https://doi.org/10.52793/jscr.2021.2(2)-S5
Abstract
In clinical medicine, hematopoietic stem cells have been utilized as a great source for disease cure and hematological advancement. Even among the great clinical capacity of these stem cells, hematopoietic stem cell transplantation has significant barriers to infant patients that receive the treatment postnatally. Alternatively, in utero hematopoietic stem cell transplantation has arisen to correct these obstacles offered by postnatal hematopoietic stem cell transplantation. In utero hematopoietic stem cell transplantation has a wide variety of advantages to the patient if a successful engraftment is achieved. Some of these benefits include the capacity to induce donor tolerance while fetal cells are immunoincompetent, a reduced need for chemotherapy and immunosuppression, and the potential to cure more diseases by early intervention. Early progress has been demonstrated in animal models; however, in human trials, the great majority of success has solely occurred in patients with immunodeficiency disorders, due to the high complexity of the fetal environment and the various ways by which the host cells may react to donor cells. In utero hematopoietic stem cell transplantation offers great promise for the future, but a wide array of clinical strategies must be further tested and investigated to truly understand how certain diseases may benefit from this new therapeutic method.
Keywords
Hematopoietic stem cells; In utero transplantation; Fetal environment; Engraftment
Introduction
Hematopoietic stem cells (HSCs) have been of great interest in recent research and clinical investigations. HSCs are multipotent cells that have the potential to create the three types of blood cells in the body: red blood cells (erythrocytes), platelets, and white blood cells (leukocytes) [1]. As they give rise to blood cells, stem cells follow the myeloid line or the lymphoid line, where the myeloid line leads to red blood cells, platelets, and myeloblasts (which form the monocytes and the granulocytes neutrophils, basophils, and eosinophils), and the lymphoid line leads to lymphoblasts (which form the lymphocytes) (Figure 1) [1]. In the fetus, HSC formation initiates during the first trimester of gestation during which the cells migrate from the yolk sac, to the liver, to the bone marrow [2]. In adults, HSCs express CD34+ and can be found in bone marrow, peripheral blood, and umbilical cord blood [1,3].
Figure 1: HSC differentiation, myeloid line and lymphoid line [1].
HSCs have the capacity to work as a standard treatment for both malignant and non-malignant disorders and diseases, and transplantations of HSCs are the only treatment to cure hematologic disorders in current time [2]. Typically, hematopoietic stem cell transplantation (HSCT) is used in pediatric cases for disorders diagnosed during gestation, and then a postnatal transplantation is performed [2]. HSCs used for transplantation can be obtained from a variety of sources: fetal liver, bone marrow, umbilical cord blood, and mobilized peripheral blood [4-5]. Transplantation may be allogeneic or autologous; an allogeneic transplantation uses HSCs from a matched donor, whereas an autologous transplantation uses the recipient’s own HSCs [6]. Fetal liver cells and bone marrow cells may be used as sources; however, these methods have presented barriers [4]. First, using fetal liver cells, the fetal donor must be at a development less than 10-12 weeks of gestation in order to avoid the risk of graft versus host disease (GVHD) [4-5]. Second, there has been significant difficulty in obtaining an adequate number of fetal donor cells to fully cure a disorder [4]. Bone marrow-derived HSCs may be obtained from a sibling’s or a parent’s bone marrow [4]. Using a parent’s bone marrow has both positive and negative aspects; fortunately, there is an unlimited supply of HSCs from such a source and T cell depletion can be easily performed [4]. Unfortunately, depleting the adult T cells can lead to a lower engraftment capability and therefore an ineffective treatment [4]. In all cases, allogeneic transplantations must find a human leukocyte antigen (HLA) match to avoid the complications of GVHD [4].
When an appropriate HSC source is obtained, the process for transplantation may occur, outlined in the following steps (Figure 2). First, stem cells are obtained from the donor, treated, and stored for preservation [6]. In the meantime, the recipient patient undergoes chemotherapy treatment so that the immune system does not fight against the incoming foreign donor stem cells [6]. Further, the HSCs are given to the recipient, the recipient is isolated, and then the recipient is analyzed upon further investigation to observe the efficacy of the transplantation process [6].
Figure 2: HSCT process [6].
A few barriers to postnatal HSCT have already been briefly discussed, but there are further considerations about the difficulties that one may encounter when undergoing HSCT. First, the fetal environment is a large hurdle to successful engraftment of the stem cells [4]. For example, GVHD is a serious complication that may result from an allogeneic transplantation [4]. In this case, the donor’s T cells will view the patient’s healthy host cells as foreign and plant an attack [7]. The only cases where such a response would not occur in a HSCT is where the donor is the recipient’s monozygotic twin, or where the donor’s T cells have been depleted from the graft [7]. Otherwise, the recipient must take medications to suppress the reactive mechanisms of the donor’s T cells which may not always be an effective strategy to prevent GVHD [7]. Another barrier to postnatal HSCT is the difficulty in obtaining a matched donor; currently for children, there is a 20-25% likelihood of finding an HLA-matched donor [4,8]. The HLA-matching process is quite complex. If the patient has an HLA identical sibling then the HSCT may be performed [8]. However, without an HLA-matched sibling, a donor must be found in bone marrow registries and cord blood banks by allele typing for eight HLA loci: A, B, C, DRB, DQA1, DQB1, DPA1, and DPB1 [8]. Under any of the following circumstances, HSCT may be performed: (1) An 8/8 matched unrelated donor, (2) An unrelated donor with cord blood matching greater than 3x107 total number of nucleated cells (TNC) per kilogram (kg) and 1-2 mismatch alleles (6/8 or 7/8 matched), or (3) A related haploidentical donor (4/8 matched) (Figure 3) [8].
Figure 3: Donor cell selection process for HSCT [9].
In addition to the infant’s environment posing complications to postnatal HSCTs, the process of HSCTs makes them somewhat undesirable. The procedure is myeloablative, meaning toxicity may result in the infant due to the high-dose chemotherapy required [4]. Myeloablation modifies the microenvironment of the recipient but is helpful because it prevents the host’s cells from competing with the donor cells during engraftment [10]. Lastly, even if an appropriate donor is found, there is often significant organ damage as a result of the patient’s disease by the time a donor is found and the transplantation becomes available after an infant’s birth [4].
Due to these barriers and the fact that there are high morbidity and mortality rates in patients who are not correctly matched to a donor, postnatal HSCT has much area to improve in the future. Alternatively, in utero hematopoietic stem cell transplantation (IUHSCT) could be utilized to treat certain disorders in fetal patients that are diagnosed in early gestation [2,10]. The goal of IUHSCT is to utilize normal hematological and immunological events that occur during fetal development in the womb to create a successful allogeneic engraftment that occurs completely in utero [2,10].
IUHSCT provides a multitude of potential advantages in comparison with postnatal HSCT. Before any transplantation is required, prenatal screening and molecular-based diagnostics that are highly specialized in current time detect diseases and disorders in the fetus during gestation [2]. A majority of congenital disorders are diagnosed by 10 weeks’ gestation, which is the optimal time in which IUHSCT should occur [10]. The main intention of IUHSCT is to incorporate transplantation in utero to cure diseases based on early interventions, so early action requires the use of prenatal screening and diagnostics (Figure 4) [2]. Primarily, the purpose of IUHSCT is to induce immunological donor tolerance in the fetus while the cells are still in utero, meaning they are still immunoincompetent [2,4,10]. Due to this immature state, the fetal cells do not immediately reject foreign antigens upon presentation because their immune cells solely consist of pre-T cells [2,10]. These pre-T cells are not alloreactive and are formed before thymic processing [2]. After thymic processing occurs, the fetus develops full T cells against foreign antigens and then deletes T cell clones against self-antigens [2]. Therefore, IUHSCT allows a nontoxic process where immunosuppression with chemotherapy before transplantation is not required as it is for postnatal HSCT [2,10]. This nonmyeloablative approach is low-intensity and avoids cytopenia, overall promoting a healthier outcome for the fetus in utero and inhibiting the need for additional postnatal treatment [2,10,11]. Further advantages of IUHSCT are that the fetal environment has circulating HSCs during gestation, which allows IUHSCT to take advantage of these normal hematological events [2]. For example, by understanding the engraftment of the host HSCs when they migrate to tissue compartments, scientists may be able to achieve a selective advantage for donor cells during engraftment [2]. The fetus typically weighs less than 35 g at 12 weeks, the optimal time for transplantation, which may give an advantage to donor cells and simply require a minimal engraftment to create a large effect [2]. Overall, IUHSCT has the potential to be extremely advantageous in preventing clinical manifestation of diseases by facilitating early intervention in utero [2,10].
Early progress for IUHSCT has been identified in animal models. The first possibility for the procedure was identified by RD Owen in 1945, where dizygotic cattle twins sharing placental circulation were observed to demonstrate mixed chimerism [2]. In other words, with mixed chimerism, bone marrow HSCs from two genetically different animals are in the same place; therefore, the two cows observed by Owen shared elements of the other twin’s blood and were immunologically tolerant of each other [2,13]. From these observations, Owen discovered that mixed chimerism may contribute to transplantation tolerance [10,13].
Figure 4: IUHSCT process [12].
Of course, mixed chimerism may be obtained naturally such as in human monozygotic twins, and there is an 8% frequency of its occurrence in human dizygotic twins; however, scientists have begun to question whether mixed chimerism can be created experimentally [2,10]. Healthy fetal sheep have been most successful at achieving experimental mixed chimerism, and they have even sustained multilineage chimerism after allogeneic transplantation of fetal liver HSCs or cord blood HSCs [10]. Unfortunately, further, scientists have found mixed chimerism difficult to replicate artificially because exposure to a twin’s blood begins very early in gestation and continues throughout a whole pregnancy [2]. Additionally, humans are much more resistant to engraftment than animal models [10].
Furthermore, animal models have found some success in trials for IUHSCTs. The first successful IUHSCT occurred in fetal murine models that lacked c-kit, leading to a stem cell deficiency and anemia in the mice [14]. On day 11 of gestation, HSCs obtained from donors’ bone marrow were injected across the placenta [14]. After direct observation, anemia was cured in the mice due to high levels of donor cell engraftment, and the host cells were completely regenerated and healthy [10,14]. Interestingly, the more severe the anemia, the more replacement of erythrocytes, indicating that engraftment is more likely achieved when there is a competitive advantage to donor cells over the host cells [2]. In another study, lymphoid regeneration was facilitated in mice with severe combined immunodeficiency (SCID) and diabetes [10]. These mice failed to develop B cells, T cells, and natural killer (NK) cells during gestation [10]. The mice models were compared: some received IUHSCT, while others received postnatal HSCT [10]. Overall, the mice treated with IUHSCT had a lower risk of GVHD, rapid regeneration of lymphoid cells, engraftment though multiple lineages, and an elevated expression of donor cells over time [10]. In further studies with allogeneic transplantations in utero, among some failure, ovine and swine models achieved multilineage chimerism, and murine models achieved both mixed chimerism and donor tolerance [2]. Animal studies have demonstrated deep clinical significance for the future of IUHSCT in humans, where most successful transplants have occurred in models with immunodeficiencies where the donor cells have a clear advantage over the host cells [2,10]. Mainly, animal studies have found that donor engraftment efficacy is difficult to obtain due to the complex, reactive microenvironment of the fetus, yet is still possible [2,10]. There are often multiple obstacles to a successful engraftment, which brings the need for further approaches and potential therapies [10].
Many trials have been conducted in humans to determine whether IUHSCT could become a standard successful treatment for various patient diseases. According to AW Flake as of 1999, there are several examples in which patients infected with SCID have been effectively cured due to IUHSCT [10]. In one SCID patient, donor HSCs obtained from the fetal liver and fetal thymus were autologously transplanted into a fetus at 28 weeks’ gestation, and upon analysis by polymerase chain reaction (PCR) assays, the HSCs were proven to obtain successful engraftment, and the patient stayed alive and healthy after birth [10]. In another SCID trial, paternal donor HSCs were enriched with CD34+ and transplanted into a fetus at 16 weeks’ gestation [10]. The patient remained alive at 40 months after birth with normal immune function, mixed chimerism, full cellular regeneration, and appropriate responses to vaccinations [10]. Finally, a SCID patient at 20 weeks’ gestation received CD34+-enriched HSCs from a paternal donor, demonstrated normal immune function at three months after birth, and exhibited mixed chimerism [10]. A more recent example of a successful IUHSCT in a SCID patient was completed by the Necker-Enfants Malades Hospital and Trousseau Hospital (part of the Paris Hospital Group) in July of 2015 [15]. The fetus was diagnosed with SCID during the first trimester of pregnancy, and at 12 weeks’ gestation, the parasite Toxoplasma gondii was transmitted from the mother to the fetus [15]. Immediate action was necessary because the parasite presented severe consequences to fetal brain development due to the fetus’ inability to fight the infection with SCID and a lack of immune cells [15]. Upon HLA analysis, the patient’s sister was perfectly matched, and HSCs and mature T cells were extracted from the sister donor then transplanted into the fetus through an intravascular infusion entering the umbilical vein at 25 weeks’ gestation [15]. A PCR assay was performed to detect the Toxoplasma gondii parasite in the amniotic fluid; the parasite was not identified, and the transplantation was deemed successful [15]. The infant was born healthy at term, with no postnatal interventions required [15]. In the previous cases, IUHSCT was performed after the first trimester of pregnancy where thymic processing has begun [2]. By evaluating SCID trials in humans, a great advantage can be identified: SCID patients that undergo IUHSCT are less likely to reject donor cells because the SCID immune system has immunoincompetency and therefore does not reject allogeneic cells [10]. The knowledge of these results may be utilized to determine whether a fetus less than 14 weeks without SCID would behave similar to the immune system of a SCID patient, in that it will not reject foreign cells because it has not completed thymic processing [4].
Another important set of diseases to consider are the hematological disorders. So far, studies on IUHSCT have been conducted in the thalassemias: alpha thalassemia and beta thalassemia. First, alpha thalassemia is a disorder in which a fetus fails at developing erythrocytes in the liver [10,11]. As a result, the fetal environment becomes hypercellular and anemic, causing a decrease in oxygen levels throughout the body due to a lack of adequate oxygen transportation [10,11]. The disorder can be detected in a few steps: the fetus produces alpha-globin-dependent hemoglobin at eight weeks’ gestation, evidence of which can be identified in an ultrasound at 10 weeks’ gestation [10]. After an ultrasound is completed, deoxyribonucleic acid (DNA) genetic sequencing is performed to confirm the diagnosis [10]. Alpha thalassemia presents danger to the fetus especially because hydrops fetalis may develop, establishing all the more reason to intervene early with IUHSCT [11]. At 10 weeks’ gestation when alpha thalassemia is diagnosed, the fetus is entering the most optimal period for IUHSCT to occur so that the disease may be cured before symptoms can even arise [10]. A few different human trials have been performed in which donor cells were injected into the peritoneal cavity, and various outcomes have evolved. One patient with alpha thalassemia received IUHSCT at 18 weeks’ gestation [10]. Upon umbilical blood sampling at 24 weeks’ gestation, no donor cell engraftment was detected, and the pregnancy was terminated [10]. However, upon autopsy, scientists were able to detect donor cell expression in multiple regions of the extramedullary hematopoiesis, which led to the discovery that engrafted donor cells may not be distinguishable until near birth time [10]. In another patient trial, IUHSCT occurred at 13 weeks’ gestation with the transplantation of paternal CD34+-enriched HSCs. The patient remained alive one year after birth, exhibited mixed chimerism, and was immunotolerant based on mixed lymphocyte reactions (MLR) [10]. Animal studies are still being performed to overcome the failures from human trials. From these, scientists have discovered that maternal HSCs have higher achievement of engraftment than any other HSC source, especially if they are CD34+-enriched and T cell depleted [3,10,11]. The most optimal period for transplantation is early in gestation so that the host cells are still immunologically immature [10]. Intravascular infusion has the capacity to create the highest engraftment levels as opposed to injections into the peritoneal cavity [11]. Most recent evidence in 2021 suggests that transamniotic delivery allows HSCs to reach the fetal environment most effectively via intra-amniotic injections, which are also the most practical and minimally invasive method [16]. For the majority, human alpha thalassemia trials with IUHSCT have not been successful and require further investigation before IUHSCT can become a standard therapy [10].
Beta thalassemia is another hematological disorder similar to alpha thalassemia; however, with beta thalassemia the patient ineffectively develops erythrocytes after birth, rather than in utero [10]. The infant produces beta-globin-dependent hemoglobin after birth, so the fetal microenvironment is viewed as normally competitive and has the capacity to react to foreign cells [10]. Several examples have investigated the impacts of IUHSCT on beta thalassemia, most of which have been autologous transplants, and so far, none of the trials have been successful at obtaining an effective engraftment in fetuses [10]. In two separate trials, autologous fetal liver HSCs were transplanted at 12 weeks’ gestation in one patient and 18 weeks’ gestation in another; both patients survived the procedure, but no engraftment was detected [10]. In another autologous trial, fetal liver HSCs were transplanted at 19 weeks’ gestation; the fetus unfortunately did not survive the transplantation to term [10]. In the final autologous trial investigated in this paper, fetal liver HSCs were transplanted at 14 weeks’ gestation; the fetus developed sepsis and the pregnancy was terminated [10]. Next, an allogeneic transplantation was completed where a matched sibling’s HSCs were depleted of T cells and transplanted into a fetus at 25 weeks’ gestation, but no engraftment was achieved [10]. Among a wide variety of trials in both animals and humans, and in a scale of disorders such as SCID, alpha thalassemia, and beta thalassemia, the majority of IUHSCT success has been limited to patients with immunodeficiencies where the donor cells have a direct advantage over the host cells [2,10].
Discussion
Among the success of IUHSCT that has been observed in animal models and the few achievements made in human trials, there still remains multiple barriers to IUHSCT that prevent the effective engraftment of donor cells [2]. First off, it has been highlighted that a major benefit of IUHSCT is its nonmyeloablative approach. However, even though this is advantageous it also provides a minor setback because the absence of chemotherapy to wipe the immune system may prevent successful engraftment [2]. Another barrier to IUHSCT is the source by which HSCs are obtained from the donor. The fetal liver is often a valuable source of HSCs; however, there are several limitations. First, HSCs for IUHSCT are only accepted from fetuses less than 10-12 weeks’ gestation [4]. Also, it may be difficult to obtain high numbers of HSCs from the fetal liver at this point in development; resultantly, engraftment in previous studies has had far lower levels than what is necessary and has been near impossible [2,4]. Another source described for IUHSCT is a parent’s bone marrow; the only limitation with this method is that the cells must be T cell depleted, which adds an extra step to the process and may impede engraftment [4].
The fetal host HSC microenvironment can be highly reactive and therefore is another obstacle to a successful engraftment [2]. Even though IUHSCT has such promise, current evidence suggests that the fetus, nonmyeloablated, may be more reactive and prevent engraftment compared to an infant that receives postnatal HSCT with myeloablation [2]. However, even though the fetus HSCs may be more reactive because there are high numbers of circulating host HSCs, there are more available niches where the engraftment may settle [10]. In other words, due to the high number of circulating HSCs in the fetus at this time of gestation, there are a multitude of niches being developed and new compartments are being enlarged for the host HSCs to inhabit [2,10]. In a positive outlook, this also provides more niches in which the donor HSCs may be able to settle and engraft, given that the donor HSCs will compete effectively with the host HSCs [2,10]. Even if the donor HSCs have equal competitiveness to the host HSCs, there is a clear advantage by which scientists can analyze the normal hematological engraftment of host HSCs in newly formed niches to understand how donor HSCs may achieve a more successful engraftment in these niches [2].
The idea of competition between host HSCs and donor HSCs within the fetal compartment has been discussed briefly. The fetal microenvironment can be highly competitive and serve as a large obstacle to the amount of engraftment that is possible [2]. Donor HSCs must be competitive in order to coexist with host HSCs [2]. The coexistence of two stem cell populations limits not only the level of engraftment but also the level of achievable mixed chimerism [2]. More specifically, fetal liver cells as well as cord blood cells are both the most competitive of all HSC sources for IUHSCT [2]. Donor cells must overcome this barrier to obtain a successful and effective engraftment that could cure a fetal disease [2]. Furthermore, competitiveness is limited by the major histocompatibility complex (MHC) of each stem cell source [10]. When the donor HSCs are MHC-matched with the host HSCs, a much more effective engraftment will occur, and the disease has a higher potential to be cured [10]. The main takeaway from scientists’ research on competition between host and donor HSCs in the fetal compartment is that host cells have the capacity to be highly reactive and may limit the level, but not the frequency, of the engraftment of donor cells and of mixed chimerism in the fetus [2].
In addition to the hurdle that the host compartment presents to IUHSCT as far as competitiveness, the host’s immune system as a whole may reject the donor stem cells [2]. During the first trimester, a fetus is generally immunoincompetent, meaning thymic processing has not yet occurred and therefore the fetus should not reject or react against any foreign cells [2]. However, if transplantation occurs after the first trimester (which may be likely, if a patient is not screened for disease and diagnosed early), then the fetal liver has already undergone thymic processing by which NK cells and T cells have fully developed and are adequate to mount an immune response [2]. Researchers are still unaware whether the fetus would respond with an innate immune response versus an adaptive immune response [2]. Therefore, immune rejection will likely occur if the transplantation is completed after the first trimester; for these reasons, early intervention is key to a successful engraftment and disease cure [2,10]. Even though the fetus should finish thymic processing and immune system development after the first trimester, scientists are still concerned by the capability of maternal immunological cells being transferred to fetal tissue [2]. Such a case would lead to maternal antibodies triggering an autoimmune response in the fetus by which the maternal T cells attack the donor cells in the fetus [2]. Due to these varied immune responses, there has only been true success for IUHSCTs in patients with an immunodeficiency disorder [2]. Further, unfortunately, even patients with SCID may be affected by the possibility of maternal cells responding to the foreign donor cells in the fetus by an autoimmune reaction [2]. Overall, the level and efficacy of an engraftment is limited by the host’s HSC competition, whereas the possibility of an engraftment occurring at all is determined by the immune response of the host’s microenvironment [2].
New developments in medicine, such as IUHSCT, must be considered for risks and consequences. IUHSCT poses risks to both the mother and the fetus during the process [10]. Fetal and maternal risks can be defined as procedural or biological [10]. Procedural risks may be identified by obstetricians before the transplantation has occurred [10]. Chorionic villus sampling (CVS) may be utilized as early as 10 weeks’ gestation, amniocentesis may be performed as early as 16 weeks’ gestation, and blood or serum sampling may be done as early as seven weeks’ gestation [10,11]. Specific procedural risks to the mother include infection, hemorrhage, and infertility [10]. Researchers have found that these risks are negligible [10]. For the fetus, the only procedural risk of true concern is possible death; however, death has occurred in less than one percent of transplants when the procedure was completed before 14 weeks’ gestation [10]. Next, one must analyze the biological risks to the fetus and the mother. In both subjects, infections from bacteria, fungi, or viruses may be transmitted from the donor cells [10]. Any case of possible infection may be easily prevented by performing tests to detect infectious disease before the donor cells are transplanted [10]. The small consequence of this process is that it adds an extra step to the IUHSCT, but one that would be worth the burden [10]. More specifically, maternal risks are quite lengthy in the biological aspect. If the donor HSCs are Rh+ and the maternal and fetal cells are both Rh-, then the mother will experience Rh sensitization and her next pregnancies will be severely affected [10]. One way to combat this risk is to ensure that Rh- mothers are receiving Rh- donor cells, or to administer Rh immunoglobulin to prevent the host cells from initiating an attack on the foreign cells [10]. Another danger to mothers is the potential for an autoimmune disease to develop if the donor lymphocytes manage to cross the placental border [10]. Researchers indicate that this event occurring is highly unlikely but may be avoided by using adult HSC sources and by depleting T cells in the donor graft [10]. The most severe threat to fetuses is GVHD, which is avoided by using adult HSC sources and executing T cell depletion in the donor cells [10].
In addition to analyzing the risks associated with IUHSCT, one must also consider the ethical consequences and applications. AW Flake indicates that there must be an ethical structure when physicians discuss the possibility of IUHSCT with their patients [10]. Counseling is often done in groups with the whole family [10]. The physician must act in a manner such that he or she is accurate, honest, and nondirective [10]. More specifically, the physician must be accurate by telling caregivers the exact details of the IUHSCT procedure without missing any important information and without skewing any data [10]. The physician must be honest by telling families all of the data on previous trials without hiding any information, and the physician must honestly explain the risks and benefits of IUHSCT [10]. Lastly, the physician must be nondirective, meaning that he or she should not use certain words to incline family decisions towards any specific therapy [10]. The physician must be deliberate in stating treatment options, while not directing caregivers toward any specific decision [10]. Another important aspect of the ethical considerations with IUHSCT is the question about the fetus’ identity [10]. For instance, the fetus is a patient, but scientists have questioned when the fetus has independent patient rights and a moral status [10]. McCullough and Chervenek determined that a fetus has a dependent moral status, meaning that a fetus’ mother must make all decisions for the fetus [10]. In addition, the mother must make these decisions autonomously, such that no other adult is able to make this decision in normal circumstances [10]. In making the decision on whether or not a fetus should undergo IUHSCT, the most essential elements to consider are the potential risks and the likely benefits that will occur due to the transplantation [10].
Now that IUHSCT has been fully described and analyzed up to date, one must consider the future directions that this therapy will take. So far, the only diseases that have truly demonstrated success with IUHSCT are immunodeficiency disorders such as SCID [2,10]. In the future with more developments, there are several more patient diseases that may benefit from IUHSCT. Most importantly, each disease must be analyzed separately and uniquely to fully determine how IUHSCT could help [10]. As earlier identified, immunodeficiency diseases are most likely to benefit from an IUHSCT therapy [10]. So far, SCID and Wiskott-Aldrich Syndrome are most promising because they offer a great advantage to donor cells over the host cells [2,10]. This means that the donor cells can be more competitive and are more likely to occupy open niches than host cells [10]. Eventually, donor HSCs will replace the host HSCs to cure the disease [2,10]. Chediak-Higashi Syndrome is another immunodeficiency disorder, but it has so far proven unsuccessful upon analysis of IUHSCT results [2].
The next class of diseases which may benefit from IUHSCT in the future are those diseases in which somatic mosaicism and spontaneous reversion occur [2,10]. Fanconi anemia and Bloom syndrome are both chromosomal breakage syndromes that may be cured by IUHSCT [10]. Adenosine deaminase deficient SCID is an autoimmune disorder in which the patient also exhibits somatic mosaicism and spontaneous reversion, so it has great potential to benefit from IUHSCT in the future [2]. This class of disease may adequately incorporate donor HSCs via an in utero transplantation because the host cells could be corrected after engraftment and then undergo clonal expansion to completely replace the host’s damaged hematopoiesis [2]. In the end, there would be a selective advantage for the cells that were spontaneously corrected [10].
Diseases that allow low engraftment levels for a large effect would certainly utilize IUHSCT as a common therapy in the future [2]. Low engraftment levels would mean that the host compartment is not particularly reactive against incoming donor cells, allowing that the donor HSCs are engrafted at high levels [2]. Examples of these disorders, although currently unsuccessful, include chronic granulomatous disease, hyper immunoglobulin M (IgM) syndrome, and leukocyte adhesion deficiency (LAD) [2].
Already previously discussed in more detail are the hematological disorders and hemoglobinopathies [2]. Alpha thalassemia has shown some miniscule success in patients, and greater success in animal models [10,11]. Beta thalassemia has demonstrated even less success. According to D Merianos, IUHSCTs for beta thalassemia almost never occur without a matched sibling donor [2]. The potential for IUHSCT in this disease would be to transplant a fetus’ parent’s HSCs in utero to overall induce tolerance in the fetus, and then incorporate those stem cells of the haploidentical parent postnatally so that the parent is an accepted donor even after birth without the use of myeloablation [2]. Lastly, sickle cell disease is a hemoglobinopathy that is so far unsuccessful upon treatment of IUHSCT amongst attempts but could show promise in the future [2].
The last class of diseases that will be discussed in this paper are the metabolic storage diseases, which include lysosomal storage disorders. At the moment, these disorders show no potential nor possibility to be cured by IUHSCT in the near future, especially because they are not even curable by postnatal HSCT [10]. In two patients in which a trial was conducted, engraftment was obtained; however, in one of the patients the disease did not cure whatsoever clinically even with successful engraftment, while the other patient suffered death due to GVHD [2].
Two unique clinical strategies may improve the future of IUHSCT and its advantages [2]. The first procedure is to utilize IUHSCT alone [2]. Such a technique would allow the fetus to obtain therapeutic levels of engraftment with one single or multiple sequential in utero transplantations [2]. At 11-14 weeks’ gestation, there is active hematopoiesis in the liver, thymic processing is at an absolute minimum, and the fetus is at a small size (less than 35 g) to allow maximum engraftment of donor cells [2]. This first strategy, as described, is favored by SCID or immunodeficiencies due to their advantage to donor cells, diseases in which somatic mosaicism occurs due to their favoring the survival of reconstituted cells, and diseases that allow low engraftment levels due to a lower competitiveness of host cells [2]. The second clinical strategy involves IUHSCT followed by postnatal conditioning with HSCT [2]. Because this strategy combines two different treatments over a longer span of time, there is high promise that this may become the standard therapeutic treatment in the future [2]. Basically, transplantation of stem cells would occur in utero, and allowing a successful engraftment at that time, further actions would be taken after the infant’s birth to strengthen the effects that were already obtained in utero [2]. This process would enhance the level of engraftment and increase tolerance to the donor cells without the need for myeloablation [2]. Scientists predict that this treatment method would be well-suited for hemoglobinopathies and hematological disorders because adequate levels of mixed chimerism may arise when healthy donor erythrocytes have a longer lifespan than the host’s diseased erythrocytes [2].
By keeping in mind the previous two clinical approaches to IUHSCT, researchers may also include the following ways by which engraftment can be improved to overall produce a more effective and therapeutic IUHSCT in patients. One way to boost engraftment is to increase the number of donor cells engrafted by elevating the receptivity of the host [2]. The non-myeloablative approach allows host cells to mobilize into circulation so that donor HSCs may more easily reach open niches and compartments for engraftment [2]. As indicated previously, engraftment is much more likely to occur in a patient that is diseased because the disabled host cells are far less competitive than the healthy donor cells [10]. So far, no studies have been performed to test this method [2]. Next, another approach is to increase the competitiveness of donor cells after one successful engraftment has already occurred [2]. This could be done by performing multiple transplants in repetitive large doses (2x109 cells/kg) [2,10]. In a fetal lamb trial, smaller doses in multiple transplants had higher engraftment levels than one large transplantation, demonstrating that there is actual replacement of host cells to create an advantage to donor cells after one successful engraftment [10]. A third way to improve engraftment capabilities is to overcome the immune barrier, but scientists are still concerned with how this would be possible without posing the risk of GVHD [2].
An intriguing concept is the idea that prenatal screening may prevent risks and complications that occur prenatally, during birth, or postnatally. A screening test determines whether a mother or fetus has a problem during pregnancy [17]. First trimester screening occurs at 11-13 weeks’ gestation and includes a maternal blood screen and an ultrasound [17]. The maternal blood sample measures levels of human chorionic gonadotropin (hCG) and pregnancy associated plasma protein A (PAPP-A) [17]. Diagnostic tests actually diagnose an issue that may have been detected in a screening test [17]. More specifically, CVS is taken at 10-12 weeks’ gestation to collect a piece of the placenta; amniocentesis is done at 15-18 weeks’ gestation to collect amniotic fluid for the fetus’ protein levels; high resolution ultrasounds are performed at 18-22 weeks’ gestation to form a picture of the fetus [17]. In 100% of the pregnancies in the United States in 2016, 77.1% of mothers received prenatal screening and diagnostics in their first trimester, 16.7% waited until their second trimester, 4.6% waited until their third trimester, and 1.6% reported none at all (Figure 5) [18]. Prenatal care has an enormous potential to improve pregnancy outcomes because it leads to early intervention and diagnosis of many diseases [2]. If all women were to undergo prenatal care in their first trimester, it is likely that diseases would have a higher chance of being detected and potentially cured by IUHSCT.
Figure 5: Initiation of prenatal care in 100% of United States pregnancies in 2016 [18].
Summary & Conclusions
In summary, IUHSCT is a very promising alternative to postnatal HSCT approaches and provides many advantages. It induces donor tolerances while the fetal cells are still in utero, it facilitates a nontoxic transplantation, it allows early treatment for diseases detected during gestation, and it overall allows for the potential cure of particular diseases by early intervention. IUHSCT has not yet achieved clinical potential in most cases, but animal models have attempted to overcome the failures from human trials and show success in animals that exhibit mixed chimerism. Transplantation of HSCs in utero is so far most successful in human patients that have immunodeficiencies such as SCID. However, one must keep in mind that even though IUHSCT overcomes several of the obstacles of postnatal HSCT, there are still multiple barriers that cause fetal engraftment to be so far unachievable in certain cases. Continuing advancements of both human and animal model studies will develop the future of IUHSCT and its possibilities. Through studies, researchers have determined that a successful IUHSCT is dependent on the gestational age of the fetal recipient, the dosage and frequency of infused HSCs, the route of administration of the HSCs, the reactivity and competitiveness of the host cell compartment, and the immunological status of the fetus. Most importantly, among the benefits of IUHSCT, one must always consider the risks that may arise to the fetus and to the mother, as well as the ethical complications that could result from IUHSCT.
References
- https://www.cancer.gov/publications/dictionaries/cancer-terms/def/hematopoietic-stem-cell
- Merianos D, Heaton T, Flake AW. (2008) In utero hematopoietic stem cell transplantation: progress toward clinical application. Biol Blood Marrow Transplant. 14(7):729-40.
- Sidney LE, Branch MJ, Dunphy SE, Dua HS, Hopkinson A. (2014) Concise review: evidence for CD34 as a common marker for diverse progenitors. Stem Cells. 32(6):1380-89.
- Cowan MJ, Golbus M. (1994) In utero hematopoietic stem cell transplants for inherited diseases. Am J Pediatr Hematol Oncol. 16(1):35-42.
- Renda MC, Maggio A. (2009) In utero haematopoietic stem cell transplantation (IUHSCT). Mediterr J Hematol Infect Dis. 1(1):31.
- https://www.mssociety.org.uk/about-ms/treatments-and-therapies/disease-modifying-therapies/hsct
- https://www.lls.org/treatment/types-of-treatment/stem-cell-transplantation/graft-versus-host-disease
- Níchonghaile M “Donor selection” ed. M Kenyon and A Babic, pp. 37-44, Springer, Cham, Switzerland, 2018
- Vogel C, personal 2021.
- Flake AW, Zanjani ED. (1999) In utero hematopoietic stem cell transplantation: ontogenic opportunities and biologic barriers. Blood. 94(7):2179-91.
- Derderian SC, Jeanty C, Walters MC, Vichinsky E, MacKenzie TC. (2015) In utero hematopoietic cell transplantation for hemoglobinopathies. Front Pharmacol. 5:278.
- Vogel C, personal (2) 2021.
- Sykes M. (2001) Mixed chimerism and transplant tolerance. Immunity 14(4):417-24.
- Fleischman RA, Mintz B. (1979) Prevention of genetic anemias in mice by microinjection of normal hematopoietic stem cells into the fetal placenta. Proc Natl Acad Sci USA. 76(11):5736-40.
- Magnani A, Jouannic JM, Rosain J, Gabrion A, Touzot F, et al. (2019) Successful in utero stem cell transplantation in X-linked severe combined immunodeficiency. Blood Adv. 3(3):237-41.
- Lazow SP, Kycia I, Labuz DF, Zurakowski D, Fauza DO. (2021) Fetal hematogenous routing of a donor hematopoietic stem cell line in a healthy syngeneic model of transamniotic stem cell therapy. J Pediatr Surg. 35:169.
- CDC, https://www.cdc.gov/ncbddd/birthdefects/diagnosis.html
- Osterman MJK, Martin JA. (2018) Timing and adequacy of prenatal care in the United States. Natl Vital Stat Rep. 67(3):1-13.
This article was originally published in a special issue entitled “Stem Cells in Regenerative Medicine”, handled by Editor. Prof. Dr. Vincent S. Gallicchio.