Evaluating Potential Stem Cell Therapies for Age-Related Macular Degeneration
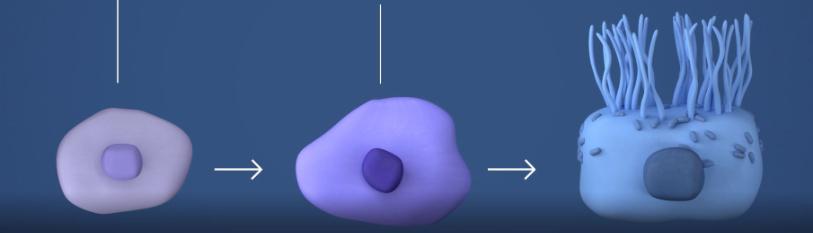
Sean Graham and Vincent S. Gallicchio*
Department of Biological Sciences, College of Science, Clemson University, Clemson, SC 29636
*Corresponding author: Vincent S. Gallicchio, Department of Biological Sciences, College of Science, Clemson University, Clemson, SC 29636
Citation: Graham S, Gallicchio VS. (2021 Evaluating Potential Stem Cell Therapies for Age-Related Macular Degeneration. J Stem Cell Res. 2(2):1-11.
Received: November 16, 2021 | Published: November 30, 2021
Copyright© 2021 genesis pub by Graham S, et al. CC BY-NC-ND 4.0 DEED. This is an open-access article distributed under the terms of the Creative Commons Attribution-Non Commercial-No Derivatives 4.0 International License., This allows others distribute, remix, tweak, and build upon the work, even commercially, as long as they credit the authors for the original creation.
DOI: https://doi.org/10.52793/JSCR.2021.2(2)-24
Abstract
The purpose of this review is to synthesize the existing literature on the intriguing research and development of stem cell treatments for age-related macular degeneration, AMD. AMD is the leading cause of vision loss for older adults across the world, and while it does not cause complete blindness, it does impact central vision, making it harder to see faces, read, drive, or do close-up work like cooking or fixing things around the house. AMD is a progressive disease that occurs in three stages, and it is subcategorized as either wet (neovascular) or dry (atrophic). There is no treatment for late-stage dry AMD, but there is a treatment for the much less common wet AMD. Pluripotent stem cells, which include human embryonic stem cells (hESCs) and induced pluripotent stem cells (iPSCs), have been used to study the development of disease processes and as potential therapies in multiple organ systems. This review focuses on the etiology of both forms of AMD, the stem cells being investigated for treatment, and the completed and ongoing clinical trials evaluating AMD stem cell treatments.
Keywords
Age-related macular degeneration; Pluripotent stem cells; Human embryonic stem cells; Induced pluripotent stem cells; Anti-vascular endothelial growth factor
Introduction
Age-related macular degeneration (AMD) is a progressive blinding disease with few treatment options and no available cure as of 2021. In 2016, it was reported that around 11 million individuals were living with AMD in the United States, and the prevalence of the disease was approximated at 170 million worldwide [1]. These statistics place AMD as the leading cause of vision loss and irreversible blindness in the industrialized world and advanced AMD as the third leading cause of visual impairment worldwide [1]. Symptoms of AMD include objects and faces appearing dark, blurry, or distorted, dark, blurry areas or whited out areas also appearing in the center of vision, and straight lines looking bent and uneven [2]. Aging is the most significant risk factor for AMD development; thus, the global prevalence of AMD is projected to increase by over 100 million cases by the year 2040 [1].
A major pathomechanism contributing to AMD development is death or dysfunction of retinal pigment epithelial (RPE) cells; RPE loss results in photoreceptor atrophy [3]. One approach being investigated to mitigate the effects of RPE loss is stem cell treatment. Human embryonic stem cells (hESCs) are pluripotent cells that give rise to all somatic cell types in the embryo. The self-renewal capacity of hESCs allows for in vitro generation of a limitless number of distinct cell types; this has prompted investigation into many novel approaches in regenerative medicine [4]. Investigating hESC-based cell therapy in the eye is favorable due to its confined and accessible nature. This allows for transplanted cells to be monitored directly in vivo, with the availability of removal or destruction if evidence of neoplastic change arises [5].
Etiology
AMD primarily affects older populations and is characterized by damage to the macula, a small area near the retina's center responsible for sharp, central vision [2]. This disease is considered a continuum of three clinical stages: early-stage AMD, intermediate AMD, and late-stage AMD. Early-stage AMD is characterized by yellowish deposits known as drusen populating the macular retina, focal hypopigmentation, or hyperpigmentation. The intermediate AMD designation is made upon identifying extensive medium drusen or at least one large druse, or both. Late-stage AMD is characterized by the presence of signs indicating either neovascular or atrophic AMD [6].
Late-stage AMD can be categorized as either wet, choroidal neovascularization, or dry, geographic atrophy AMD [7]. The result of end-stage wet AMD is permanent loss of central vision. Sustained progressive damage to Bruch's membrane in conjunction with an increase in expression of vascular endothelial growth factor (VEGF) stimulates the projection of abnormal choroidal vessels underneath the Human retinal pigment epithelium (RPE), eventually protruding under the retina [7]. Subretinal extravasations found in these irregular vessels present the possibility of hemorrhage, resulting in the formation of disciform scars and loss of central vision [7]. Bruch's membrane is a five-layered extracellular matrix that lies between the retinal pigment epithelium (RPE) and choroid of the eye. This structure is involved in mediating interactions between the retina and general circulation, including the exchange of biomolecules, nutrients, and oxygen [8].
RPE undergoes various changes during aging, leading to the emergence of a clinically detectable focal yellow accumulation of extracellular, polymorphous material, drusen, at the interface between the RPE and the inner collagenous zone of Bruch's membrane [9]. Accumulation of drusen in this area of Bruch's membrane can lead to calcification and fragmentation. Abnormalities such as these have been shown to impair RPE cell reattachment, survival, and proliferation in ex vivo culture systems that model AMD [9]. Anti-vascular endothelial growth factor (anti-VEGF) intravitreal injections are the current Food and Drug Administration (FDA)-approved treatment for neovascular, wet AMD. Despite these treatments being effective in treating the complications associated with AMD, they do not reverse the damage caused prior to treatment [10].
One pathway investigated for the underlying causes of dry AMD begins with RPE death which leads to photoreceptor loss, subsequently leading to visual loss over time. Low levels of DICER1, a ribonucleic acid (RNA)-cleaving enzyme, have been observed in the RPE cells of patients with dry AMD [7]. Al-Zamil et al. reported that lower levels of DICER1 lead to a reduced rate in the breakdown of RNA-Alu molecules, which are non-coding sequences of RNA. Excessive amounts of cytoplasmic RNA-Alu activate inflammatory proteins, such as NLRP3 in the inflammasome, which activate a cascade of molecular reactions, resulting in RPE cell death [7]. Additionally, RPE death and the development of dry AMD have been associated with mitochondrial dysfunction. Al-Zamil et al. noted that in the RPE of individuals with AMD, mitochondrial dysmorphology consistent with severe mitochondrial dysfunction has been observed [7].
Risk factors for AMD include but are not limited to being a member of the Caucasian race, smoking, and family history of developing AMD. The prevalence of AMD in White populations was more than double that of Black, Hispanic, or other races in 2010 [1]. One study completed in the United States in 2014 found that African Americans, Latinos, and Asian Americans were 19% to 74% less likely to be diagnosed with AMD, and these groups were 48% to 86% less likely to receive an anti-VEGF intravitreal injection for AMD when compared to White Americans [11]. Smoking cessation is considered by most researchers to be the most important modifiable risk factor for AMD development and progression [12]. Many cross-sectional studies have been completed showing that smoking has been the most consistent factor associated with geographic atrophy and neovascular AMD [12].
Due to the significant involvement between the complement system and the innate immune system in the development of AMD, complement factor H (CFH) has been investigated as a key molecular player in the change from normal RPE aging into AMD pathology [7]. CFH suppresses the complement pathway; thus, abnormal CFH activity causes the complement cascade to be activated, causing a downstream inflammatory response to subretinal tissues [7]. Within the drusen observed in cases of AMD, inflammatory components from the complement cascade pathway have been found. Smoking is associated with a decrease in CFH levels, which may explain the significant increase in risk for developing AMD among smokers when compared to nonsmokers [7].
The complement pathway is a major part of the human innate immune system, consisting of many distinct plasma proteins that induce a series of inflammatory responses that help fight infection [13]. There are three pathways in the complement system: the classical pathway, the lectin pathway, and the alternative pathway. The alternative pathway is regarded as the amplification mechanism for the other two pathways and is considered the pathway most closely linked to the pathogenesis of AMD [14]. Currently, the extent of the complement system's role in AMD pathogenesis remains unclear [14].
Epidemiology
The risk for developing early-stage and late-stage AMD is linked to multiple variables, including age, genetics, smoking, nutrition, diet, sunlight exposure, body mass index, and cardiovascular disease [15]. Based on a meta-analysis completed by Jonas et al. in 2017, within the age of 45-85 years, the global prevalence of any AMD, early AMD, and late AMD were 8.7%, 8.0%, and 0.4%, respectively. Additionally, this group found that early AMD was less common in those of Asian ancestry (6.8%) than in Europeans (11.2%). The prevalence of late AMD did not differ significantly between Asians and Europeans, and AMD of any stage was less common in individuals of African ancestry [16]. The global prevalence of any type of AMD was approximately 8.7%, with the prevalence of early AMD being 8.0% and the prevalence of late AMD being 0.4% [16]. Jonas et al. did not find sex to be significantly associated with the prevalence of AMD or with the frequency of AMD as a cause for vision impairment or blindness [16].
Incidence of Disease
The prevalence of AMD in the U.S. is like that of all invasive cancers combined and more than double the prevalence of Alzheimer’s disease [1]. In 2016 it was estimated that about 11 million individuals live with AMD in the United States. This number is expected to increase to 22 million by the year 2050 in part due to an aging population [1]. The global prevalence of AMD is estimated at 170 million, and this number is expected to increase to 288 million by the year 2040 [1]. Additionally, hypertension and high BMI are associated with an increased risk of the development of AMD [1]. Conversely, it has been reported that physical exercise is a significant protective factor against the incidence of geographic atrophy, neovascular AMD, and progression of AMD [1].
Morbidity and Mortality
There is currently no conclusive evidence linking AMD and mortality; however, evidence has been found to associate AMD with cardiovascular and inflammatory diseases. From a McGuinness et al. meta-analysis of 12 reports and 10 studies, late-stage AMD was associated with elevated rates of all-cause and cardiovascular mortality; however, early AMD was not. Additionally, there was no evidence of an association between early or late AMD and cancer mortality [17]. Similar meta-analysis studies have been completed with corresponding findings when those with AMD were compared to those who did not have AMD and were of similar age and sex [18].
Current Standard of Care
For individuals who develop advanced AMD, the availability of treatment depends on whether they have the wet or dry form of the condition. There is no FDA-approved treatment for dry AMD as of October 2021. Care for wet AMD includes multiple approaches to arrest and reverse the effects of Choroidal Neovascularization in the macula. The current standard of care is the injection of anti-VEGF medications into the eye's vitreous cavity. Anti-VEGF treatments work by inhibiting the growth of abnormal new blood vessels [19].
Although various molecular pathways have been implicated in AMD, the primary focus of the current treatment strategy is to modulate the complement cascade. VEGF inhibitors, such as ranibizumab, aflibercept, and bevacizumab, are administered intravitreally to limit exudation from the leaky vessels and improve retinal morphology [15]. Treatment is administered monthly, and the initiation of therapy usually consists of 3 doses. Chakravarthy and Peto report that cessation of treatment can result in recurrence of exudation; there is no current guidance about when treatment might be stopped, and treatments fail to affect the underlying disease mechanisms [15]. Low vision aids and rehabilitation strategies are still currently employed to support patients who become bilaterally visually impaired due to late-stage AMD.
Additional therapies are aimed at controlling the disease and preserving vision for years or longer after detection. Thus, early detection, smoking cessation, and a healthy diet are crucial to reducing the risk of progression to advanced AMD and preventing related vision loss. Currently, there are ways for patients to monitor their vision at home, such as an Amsler grid (a grid of horizontal and vertical lines used to monitor the central visual field), and more advanced home monitoring systems, such as retinal scanning devices, are expected in the near future [19].
Current areas of unmet need in the treatment of geographic atrophy due to AMD are the lack of effective treatments to prevent progression from the intermediate AMD stage. For those suffering from dry AMD, clinicians' only recourse is early diagnosis and advising steps to slow the disease's progression, such as taking vitamin supplements, eating healthfully, and not smoking [20].
Gene Therapy
Focusing on curtailing complement activation, which plays a role in AMD progression, recently, there has been a surge in new immune-modulatory strategies. However, currently approved genetic treatment options remain scarce despite many promising in vitro findings from clinical trials. [14]. The current understanding of the genetic pathology of AMD is incomplete and in need of further research. Despite the promising work and findings of research teams worldwide, gene therapy retains significant challenges for its treatment application. Dr. Osama Sabbagh, MD et al. say that one of the most pressing issues regarding gene therapy lies in the vector’s delivery. AAV vectors are the most common form of delivery used in research due to their many advantages; however, this method has shown the troublesome ability to transduce cells, particularly when given intravitreally [21]. Additionally, the current capacity of AAV vectors is limited at approximately 4.7 kb [21]. According to Sabbagh et al., subretinal delivery may be more effective than the use of AAV vectors, but the risk of damage to the retina and cataract formation persists [21]. The long-term effectiveness of gene therapies remains up for debate, and many researchers have voiced concerns over the possible effects of continuous, sustained suppression of VEGF [21]. Due to the difficulty of manufacturing current gene treatments, Sabbagh reports that gene therapy remains expensive and may create a burden for patients and the healthcare system [21].
Types of Stem Cells being Investigated
Human embryonic stem cells (hESCs) present researchers with the promise of cellular replacement therapies due to their availability, pluripotency, and unlimited self-renewal capabilities [5]. However, these cells are also susceptible to neoplastic change, uncontrolled proliferation, and differentiation to harmful cell types [5]. Current wet AMD therapies include administering angiogenesis inhibitors and retinal translocation surgery [5]. Focusing solely on suppressing the disease, these wet AMD treatments require long-term repeat delivery and their transplantation counterpart, although providing restoration of macular anatomy, does not prevent disease recurrence [5]. One method being investigated to solve this problem is the use of human embryonic stem cell (hESC)-derived retinal pigment epithelium (RPE) cells in a patch form. These patches attempt to supply the eye with cells that are effectively zero years old, rather than those the age of patients which contain the predisposition to develop AMD [5]. Despite hESC-derived RPE cell patches representing a promising future, further research is needed to address their use in achieving the goal of curing AMD.
Induced pluripotent stem cells are a type of pluripotent stem cell derived from adult somatic cells that have been genetically modified through the forced expression of specific genes and factors to an embryonic stem cell-like state [22]. Using iPSCs, researchers obtain pluripotent stem cells that are not controversially derived from embryos and provide an effective way to “de-differentiate” cells [22]. iPSCs present researchers with the potential of generating all tissues of the human body as well as patient and disease specific cells that could be used for treating diseases such as AMD [22].
Additionally, human mesenchymal stem cells are the focus of research for the treatment of degenerative retinas, including those diseased by AMD. Human mesenchymal stem cells can differentiate into the various mesenchymal tissues such as osteoblasts, chondrocytes, and adipocytes [10]. Due to their pluripotent nature and studies demonstrating a possible protective association between human mesenchymal cells and damage related to AMD, these stem cells are being investigated for use as local immunomodulatory agents.
Animal Models
Studies investigating the efficacy of hESC-derived RPE cells have been conducted in mice and pigs. Qu et al. investigated the effects of combined human mesenchymal stem cell and human retinal progenitor cell subretinal transplant in rats. Upon completion of this combined therapy, Qu et al. reported improvement in electroretinogram performance and outer nuclear layer thickness, increased migration of grafted cells, and reduced activation of microglia and Müller cell gliosis compared to single transplantation of human progenitor cells or human mesenchymal stem cells. Both human mesenchymal stem cells and retinal progenitor cells have been transplanted clinically for the treatment of different retinal diseases, but the results of this study suggest that more satisfactory outcomes may be possible if combination therapy with both types of cells is administered [10,23].
Additionally, human mesenchymal cells have been shown to protect against RPE cell degeneration in rats and rabbits [24]. In these in vivo models, mesenchymal stem cells have prevented damage from NaIO3, a model for geographic atrophy. Following treatment of NaIO3, researchers observed increased mRNA levels for genes associated with the development of AMD [24]. When the administration of mesenchymal stem cells followed NaIO3 treatment, retinal cell degeneration was not observed. These findings are promising as the degeneration of RPE cells is one of the first steps in the progression of AMD, meaning mesenchymal stem cells may be an option for treating this disease [24].
Induced pluripotent stem cells have demonstrated the ability to differentiate into photoreceptors and RPE cells and integrate into the host cell structure. Induced pluripotent stem cell therapies have significantly improved retinal function in retinal dystrophic and degenerative rat and mouse models. MacLaren et al. observed that rod photoreceptor precursors derived from the post-natal day one retina of mice can be incorporated into the outer nuclear layer of the mammalian retina, improving vision in mouse models of degenerative retinal disease [10,25]. Additionally, it has been demonstrated that transplanted photoreceptor cell suspensions, including iPSCs, can restore visual function in animal models of retinal degeneration [26].
Clinical Trials
Mandai et al. completed a clinical study initiated in 2013, testing the feasibility of transplanting an iPSC-RPE sheet in a patient with neovascular, wet, age-related macular degeneration. Patient one, a 77-year-old Japanese woman, received a diagnosis of polypoidal choroidal vasculopathy (a subtype of neovascular AMD) in both eyes in 2010. She had received regular injections of an anti-VEGF drug, as clinically indicated, over 29 months, but the best-corrected visual acuity in her right eye gradually declined to 0.09 (lower than 20/200 on a Snellen chart). In Patient 1, the neovascular membrane was removed, and an autologous iPSCs derived RPE cell sheet was transplanted. No serious adverse event was noted after 25 months in this patient, they did not receive immunosuppressants, and no signs of rejection were observed. After one year, the best-corrected visual acuity of the treated eye had not improved or worsened, and her Visual Functioning Questionnaire score had improved from 48.8 (before surgery) to 58.3 (one year after surgery). However, Mandai et al. recognize it is possible that this outcome could have been obtained without surgery. Her score on Optical coherence tomographic images indicated good retinal integrity over the graft one year after the transplantation, with a high-density area, which may indicate an area of recovering inner and outer segments of photoreceptor cells. The researchers were unable to evaluate the extent of the photoreceptor function of the RPE graft in this patient [27]. No genomic aberrations suggestive of tumorigenicity were observed in the iPSCs derived RPE cells obtained from Patient 1. The findings of this study are limited due to only a single patient receiving treatment; however, the approach of this study has been expanded upon in recent years due to its promising and informed approach [27].
Dysfunction and loss of retinal pigment epithelium are central to disease progression in wet AMD. One strategy investigated to circumvent this progression is the surgical implantation of an engineered RPE patch comprising a fully differentiated, hESC-derived RPE monolayer on a coated, synthetic basement membrane [5]. Using a purpose-designed microsurgical tool, researchers implanted this patch into the subretinal space of one eye in each of two patients with severe wet AMD. Da Cruz et al. reported successful delivery and survival of the RPE patch by biomicroscopy and optical coherence tomography, and a visual acuity gain of 29 and 21 letters in the two patients treated in the trial, respectively, over 12 months. Da Cruz et al. claim that the results of this trial support the viability and safety of the transplantation of hESC-derived RPE patches as a treatment for AMD [5].
In 2021, Unity Biotechnology announced positive results from their phase-1 clinical trial of UBX1325 in advanced vascular eye disease. This Phase 1 safety study of UBX1325 includes patients with wet AMD for whom anti-VEGF therapy was no longer considered beneficial. UBX1325 is a small molecule inhibitor of Bcl-xL and researchers hope to use this senolytic drug to target ophthalmologic diseases by utilizing a biologic pathway distinct from anti-VEGF therapies. Unity Biotechnology reports that this treatment has been well-tolerated with no treatment-related adverse events or dose-limiting toxicities up to 12 weeks. Their data show strong and sustained responses following a single injection of UBX1325 out to 12 weeks. Additionally, UBX1325 maintained responses in crucial clinical measures of disease progression in most patients through 12 weeks, including substantial improvements in best-corrected visual acuity and central subfield thickness. The 24-week data from this study is expected before the end of 2021 [28].
In two prospective phase I/IIa studies, Schwartz et al. evaluated the safety and tolerability of subretinal transplantation of hESC-derived retinal pigment epithelium in nine patients with Stargardt’s macular dystrophy and nine with dry AMD [29]. Three dose cohorts were treated for each eye disorder, and all patients who received transplants were assessed regularly for a median of 22 months using serial systemic, ophthalmic, and imaging examinations. Schwartz et al. report that during this trial, there has been no evidence of adverse proliferation, rejection, or serious ocular or systemic safety issues related to the transplanted tissue [29]. Additionally, best-corrected visual acuity improved in ten eyes, improved or remained the same in seven eyes, and decreased by more than ten letters in one eye. In the untreated fellow eyes, similar improvements in visual acuity were not observed [29]. In patients with dry AMD, vision-related quality-of-life measures increased for general and peripheral vision and near and distance activities. These findings represent evidence for the safety of hESC-derived cell therapies in humans and the possibility of graft survival within the human eye. These promising outcomes suggest that hESC-derived cells could be used to treat various disorders and diseases requiring tissue repair or replacement, including dry AMD [29].
OpRegen® is a cell-based product composed of RPE cells, derived from hESCs and administered as a cell suspension either in ophthalmic Balanced Salt Solution Plus or in CryoStor® 5 (Thaw-and-Inject, TAI). This product is being investigated for its potential use in treating dry AMD. Lineage Cell Therapeutics, Inc. is completing a clinical study evaluating the safety and tolerability of OpRegen as well as exploration of the ability of transplanted OpRegen cells to engraft, survive, and moderate disease progression. Specifically, this study explores the safety and tolerability of OpRegen transplantation in patients with progressive dry AMD. The subjects are 50 years of age and older, with non-neovascular, dry AMD, who have funduscopic findings of geographic atrophy in the macula, with the absence of other concomitant ocular disorders. This study began in 2015, and its estimated study completion date is December 2024. As of October 2021, no data has been posted [30].
In 2020, The National Eye Institute launched a clinical trial to test the safety of a novel patient-specific stem cell-based therapy to treat advanced dry AMD. The therapy involves converting a patient’s blood cells into iPSCs modified to develop into RPE cells. In order to promote the integration of these cells into the retina, they are grown in sheets one cell thick. Then this monolayer is grown on a biodegradable poly lactic-co-glycolic acid (PLGA) scaffold and assessed for plausibility as an autologous cell-based therapy for geographic atrophy associated with AMD [31,32]. Twelve patients with advanced dry AMD will receive the iPSC-derived RPE implant in one of their eyes. Researchers will then monitor recipients for at least one year to confirm safety. By replacing dying retinal cells with iPSCs derived RPE, this therapy attempts to strengthen the recipients’ remaining photoreceptors. Additionally, this ongoing trial awaits the Food and Drug Administration’s designation of iPSC-derived RPE as a clinical-grade product. As of October 2021, no data has been posted for this clinical trial. [31,32].
Astellas Institute for Regenerative Medicine is currently completing a clinical study evaluating the safety and feasibility of a new treatment called ASP7317. This study aims to investigate the slowing or reverse of atrophy in dry AMD. ASP7317, which consists of specially created cells derived from human stem cells, is injected into the eye’s macula while the subject is under anesthesia. An immunosuppressive medicine (tacrolimus) is also taken around the time of injection of the cells with the goal of preventing cell rejection from the body. As of October 2021, no data has been posted for this clinical trial. This study’s estimated primary completion date is November 30, 2022 [33].
Conclusions
Age-related macular degeneration is a global issue, affecting around 170 million people worldwide, and yet there is no accepted cure for this disease and few to no treatment options available depending on individual circumstances. As the world population ages, the number of people at greatest risk for developing AMD shall grow immensely. While promising, hESC and iPSC therapies have a long way to go before comprehensive AMD treatments are available for patients. It is imperative that institutions, researchers, and governing bodies prioritize the research and development of AMD treatment now so that we may avoid the impact and ramifications of the projected increase to 288 million AMD cases worldwide by 2040.
References
- Pennington KL, DeAngelis MM. (2016) Epidemiology of age-related macular degeneration (AMD): Associations with cardiovascular disease phenotypes and lipid factors. Eye and Vision. 3[1].
- https://www.nei.nih.gov/learn-about-eye-health/outreach-campaigns-and-resources/eye-health-data-and-statistics/age-related-macular-degeneration-amd-data-and-statistics
- Mazzilli JL, Snook JD, Simmons K, Domozhirov AY, Garcia CA, et al. (2020) A preclinical safety study of human embryonic stem cell-derived retinal pigment epithelial cells for macular degeneration. J Ocul Pharmacol Ther. 36(1):65-9.
- Vazin T, Freed,WJ. (2010) Human embryonic stem cells: Derivation, culture, and differentiation: A Review. Restor Neurol Neurosci. 28(40:589-603.
- da Cruz L. Fynes K, Georgiadis O, Kerby J, Luo YH, et al. (2018) Phase 1 clinical study of an embryonic stem cell–derived retinal pigment epithelium patch in age-related macular degeneration. Nature Biotechnol. 36(4):328-37.
- Waugh N, Loveman E, Colquitt J. (2018) Treatments for dry age-related macular degeneration and Stargardt disease: a systematic review. Southampton (UK): NIHR Journals Library; 2018 May. (Health Technology Assessment, No. 22.27.) Chapter 1, Introduction to age-related macular degeneration.
- Al-Zamil W, Yassin S. (2017) Recent developments in age-related Macular degeneration: A review. Clin Interv Aging. 12:1313-30.
- Booij JC, Baas DC, Beisekeeva J, Gorgels TGMF, et al. (2010) The dynamic nature of Bruch's membrane. Prog Retin Eye Res. 29(1):1-18.
- Gong J, Cai H, Noggle S, Paull D, Rizzolo LJ, et al. (2019) Stem cell‐derived retinal pigment epithelium from patients with age‐related macular degeneration exhibit reduced metabolism and matrix interactions. STEM CELLS Translational Med. 9(3):36-76.
- Ludwig PE, Freeman SC, Janot AC. (2019) Novel stem cell and gene therapy in diabetic retinopathy, age related macular degeneration, and retinitis pigmentosa. Int J Retin Vitr. 5[1].
- Mahr MA, Hodge DO, Erie JC. (2018) Racial differences in age-related macular degeneration and associated anti–Vascular Endothelial Growth Factor Intravitreal injections among Medicare beneficiaries. Ophthalmol Retina. 2(12):1188-95.
- Velilla S, García-Medina JJ, García-Layana A, Dolz-Marco R, Pons-Vázquez S, et al. (2013) Smoking and age-related macular degeneration: Review and update. J Ophthalmol. 2013:1-11.
- Janeway CA, Jr. (2001, January 1). The complement system and innate immunity. Immunobiology: The Immune System in Health and Disease. 5th edition.
- Wu J, Sun X. (2019) complement system and age-related macular degeneration: Drugs and challenges. Drug Des Devel Ther. 13: 2413-425.
- Chakravarthy U, Peto T. (2020) Current perspective on age-related macular degeneration. JAMA. 324(8):794.
- Jonas JB, Cheung CMG, Panda-Jonas S. (2017) Updates on the epidemiology of age-related macular degeneration. Asia-Pacific J Ophthalmol.
- McGuinness MB, Karahalios A, Finger RP, Guymer RH, Simpson JA. (2017) Age-related macular degeneration and mortality: A systematic review and meta-analysis. Ophthalmic Epidemiol. 24(3):141-152.
- Xin X, Sun Y, Li S, Xu H, Zhang D. (2018) Age-related macular degeneration and the risk of all-cause and cardiovascular mortality. Retina. 38(3):497-507.
- https://www.health.harvard.edu/blog/age-related-macular-degeneration-early-detection-and-timely-treatment-may-help-preserve-vision-2020112421362
- Pruthi S. (2021, May 8). Dry macular degeneration. Mayo Clinic.
- Sabbagh O. (2020, March 21). Gene therapy in AMD: Promises and challenges. Retina Specialist.
- Ye L, Swingen C, Zhang J. (2013) Induced pluripotent stem cells and their potential for basic and Clinical Sciences. Current Cardiol Reviews. 9(1):63-72.
- Qu L, Gao L, Xu H, Duan P, Zeng Y, et al. (2017) Combined transplantation of human mesenchymal stem cells and human retinal progenitor cells into the subretinal space of RCS Rats. Scientific Reports, 7[1].
- Holan V, Palacka K, Hermankova B. (2021). Mesenchymal stem cell-based therapy for retinal degenerative diseases: Experimental models and clinical trials. Cells. 10(3):588.
- MacLaren RE, Pearson RA, MacNeil A, Douglas RH, Salt TE, et al. (2006). Retinal repair by transplantation of photoreceptor precursors. Nature. 444(7116):203-07.
- Chichagova V, Hallam D, Collin J, Zerti D, Dorgau B, et al. (2018) Cellular regeneration strategies for Macular degeneration: Past, present and future. Eye. 32(5):946-71.
- Mandai M, Watanabe A, Kurimoto Y, Hirami, Morinaga C, et al. (2017). Autologous induced stem-cell–derived retinal cells for macular degeneration. New England J Med. 376(11):1038-1046.
- https://ir.unitybiotechnology.com/news-releases/news-release-details/unity-biotechnology-announces-positive-12-week-data-phase-1/
- Schwartz SD, Regillo CD, Lam BL, Eliott D, Rosenfeld PJ, et al. (2015) Human embryonic stem cell-derived retinal pigment epithelium in patients with age-related macular degeneration and Stargardt's macular dystrophy: Follow-up of two open-label phase 1/2 studies. The Lancet. 385(9967):509-16.
- Lineage Cell Therapeutics, Inc. (2021, July 27). Safety and efficacy study of opregen for treatment of advanced dry-form age-related macular degeneration - full text view. Full Text View - ClinicalTrials.gov.
- DeMott K. (2019). NIH launches first U.S. clinical trial of patient-derived stem cell therapy to replace dying cells in Retina. National Institutes of Health.
- National Eye Institute. (2021). Autologous transplantation of induced pluripotent stem cell-derived retinal pigment epithelium for geographic atrophy associated with age-related macular degeneration
- Astellas Institute for Regenerative Medicine. (2021). A study of the safety and tolerability of asp7317 in adults who are losing their clear, Sharp Central Vision due to geographic atrophy secondary to dry age-related macular degeneration.