Dental Stem Cell Banking and Applications of Dental Stem Cells for Regenerative Medicine: A Literature Review
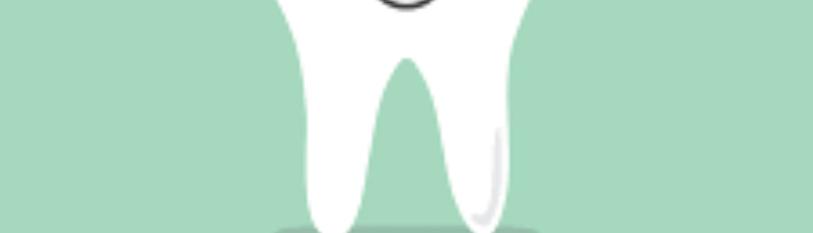
Karley D Bates and Vincent Gallichio*
Department of Biological Sciences, College of Science, Clemson University, Clemson, South Carolina, USA
*Corresponding author: Vincent S Gallicchio, Department of Biological Sciences, College of Science, Clemson University, Clemson, South Carolina,USA
Citation: Gallicchio VS, Bates KD. (2020) Dental Stem Cell Banking and Applications of Dental Stem Cells for Regenerative Medicine: A Literature Review. J Stem Cell Res. 1(2):1-10.
Received: October 03, 2020 | Published: October 30, 2020
Copyright© 2020 genesis pub by Bates K, et al. CC BY-NC-ND 4.0 DEED. This is an open-access article distributed under the terms of the Creative Commons Attribution-Non Commercial-No Derivatives 4.0 International License., This allows others distribute, remix, tweak, and build upon the work, even commercially, as long as they credit the authors for the original creation.
DOI: https://doi.org/10.52793/JSCR.2020.1(2)-10
Abstract
Since the identification of mesenchymal stem cells, stem cell biology is a greatly researched field of regenerative medicine and tissue engineering therapies and has become an essential part of practical dentistry. Mesenchymal stem cells are multipotent stem cells that can differentiate into many cell types. Dental mesenchymal stem cell populations have been identified in dental pulp, human exfoliated deciduous teeth, periodontal ligament and dental follicle of third molars and tooth germ of third molars, gingival of periodontium, alveolar bone, and apical papilla. Dental stem cells are the most natural, noninvasive source of stem cells that have been identified, and they have gained recent attention due to their accessibility and the associated relatively low cost of integration into regenerative therapy. Long-term preservation of dental stem cells is becoming a popular consideration and mirrors the ideology of banking umbilical cord blood. This review outlines the recent progress in the mesenchymal stem cells used in dentistry as well as some advancement that are being made in preserving dental stem cells for future personalized medicine. The aim of this study was to completely and concisely review the current use of adult dental stem cells specifically oral sources of stem cells, banking of dental stem cells, and applications or uses of dental stem cells specifically in oral regions and in a clinical setting.
Keywords
Dental mesenchymal stem cells; Stem cell banking; Regenerative dentistry
Abbreviations
MSCs: Mesenchymal Stem Cells; SHED: Stem Cells from Human Exfoliated Deciduous Teeth; DPSC: Dental Pulp Stem Cells; PDL: Periodontal Ligament; PDLSC: Periodontal Ligament Stem Cells; DFSC: Dental Follicle Stem Cells; TGPC: Tooth Germ Progenitor Cells; GMSC: Gingival Derived Mesenchymal Stem Cells; ABMSC: Alveolar Bone Mesenchymal Stem Cells; SCAP: Stem Cells from the Apical Papilla; BMMSC: Bone Marrow Derived Mesenchymal Stem Cells; DMSC: Dental Derived Mesenchymal Stem Cells; HATCP: Hydroxyapatite/Tricalcium Phosphate
Introduction
There exists an association between oral health and overall health throughout the lifetime of an individual. Because of this association, advancements are being made in dental practice to maintain teeth and orofacial bone as a way to combat general decline in oral health. Current common practice includes the use of artificial materials in dental implant procedures and other prosthodontic therapies. While often effective, these methods frequently require costly and invasive procedures and may result in failure or rejection. Recent research has given much attention to bettering therapeutic treatment and regenerative medicine associated with maintaining oral health. At the forefront of this research is investigating the use of stem cells in tissue regeneration, specifically in dental practices [1]. The use of stem cells in dentistry focuses on making the transition from using artificial materials in treatments to using individualized biological materials resulting in greater personalized medicine. The oral cavity has many locations from which to obtain stem cells, and stem cells can be harvested throughout many stages in an individual’s life. Stem cell preservation through banking must be considered as a preventative way to obtain the biological materials necessary to make personalized medicine a common practice.
Adult stem cells, specifically mesenchymal stem cells (MSCs), can be loosely defined as immature multipotent cells that mature and multiply with the capacity to develop into many and more specific types of cells and tissues through the process of differentiation. They are derived from neural crest cells and MSCs during embryonic and child development [2]. A cell must be able to self-replicate and differentiate into two or more cell types to be considered MSCs [3]. Stem cells are the greatest source for means of regenerative medicine because they are autologous to the native tissue with low tumorigenesis risk and unlikely immune rejection [4]. Tooth derived MSCs, from both primary and permanent teeth are of great interest regarding regenerative medicine because of how accessible they are due to the minimally invasive means through which these stem cells are obtained.
Stem cell use in dentistry is on the forefront of research and clinical application because there are many sources of stem cells within the oral cavity. Clinical treatment of a number of dental, oral, and craniofacial diseases using stem cells is currently being explored. Tooth-derived MSCs have great capability to proliferate and differentiate into many mesoderm lineages, and their performance aligns with MSCs from non-oral origins [5].
New discoveries continue to be made regarding which stem cells have the most advantageous characteristics for success in allogenic applications in dental regenerative medicine. Dental stem cells come from a number of sources that can be obtained at different points throughout an individual’s life. These sources include dental pulp [6], human exfoliated deciduous teeth (SHED) [7], periodontal ligament of periodontium [8] and dental follicle of third molars [9], tooth germ progenitor cells of third molars [10], gingiva of periodontium [11], alveolar bone [12], and apical papilla [13-15]. Each of these sources has varying levels of cell proliferation and a range of differentiation capabilities.
Two types of stem cells are necessary for dental hard tissue regeneration: epithelial stem cells, which are responsible for the formation of enamel, and mesenchymal stem cells, which are responsible for the formation of dentin [16]. While research and clinical applications exist regarding MSCs use in dentistry, no information is currently available regarding dental epithelial stem cells [1,16].
The future success of stem cell therapy will be exemplified through a process known as stem cell banking, which includes harvesting, storing, and cryopreserving the cells. Stem cell banking highlights the ideology of individually personalized medicine being the best preparation for future treatment and is quickly increasing in research and clinical attention [17]. Advantages to dental stem cell banking include: (a) safety in harvesting, (b) low ethical concerns present in compared to that of applications of embryonic stem cells [3], and (4) non-invasive means of harvesting [7], and autologous source of stem cells.
Background
MSCs are the group of stem cells that are the most researched and the stem cell type most commonly used in clinical applications. Being multipotent, they possess the capability to mature and differentiate into multiple mesenchymal tissue lineages: mesodermal, ectodermal, and endodermal lineages [18]. MSCs are also characterized by their ability to self-renew and proliferate to maintain the source of undifferentiated stem cells. Additionally MSCs have been shown to possess immunomodulatory effects including angiogenesis, anti-inflammation, and anti-apoptosis [1] showing their great potential for use in regenerative medicine. Furthermore, MSCs are associated with minimal ethical controversy and can be obtained from many adult tissues in the body [17-19]. The study of human MSCs began with their identification present in bone marrow. Through experimentation, bone marrow derived stem cells (BM-MSCs) have been found to regenerate certain skeletal tissues including bone, cartilage, and adipose and fibrous tissues [20]. While research has shown effective use of BM-MSCs in tissue regeneration, the rather invasive measures through which these cells must be obtained make BM-MSCs an unlikely candidate for expansion and normalization of personalized medical treatments. Scientists since have investigated methods to obtain and isolate MSCs from other various tissues in the body. Some of these include adipose tissue [21], endometrial tissue [22], salivary gland [1], umbilical cord [23], synovial membrane and fluid [24], blood [25], and oral tissues [1]. MSCs from oral sources are among the most easily accessible stem cells and often may be obtained through minimally invasive measures. They have also been found to possess similar immunomodulatory characteristics as BM MSCs [26]. Because of their accessibility, researchers continue to investigate their clinical applications in regenerative medicine. There are eight identified populations of MSCs present in teeth and their supporting structures of the oral cavity.
Dental Pulp Stem Cells
In 2000 Gronthos et al. [6] were the first to identify MSCs located in the dental pulp of teeth that are today known as dental pulp stem cells (DPSC). These cells were investigated based on their supposed clonogenic and proliferative characteristics. Gronthos et al. [6] suggested that DPSC have the progenitors required to amount similar cellular characteristics to those of BM-MSCs, a population of stem cells that is better understood. The research focused on comparing DPSC forming odontoblasts and BM-MSCs forming osteoblasts, specifically investigating if the DPSC possess the same proliferation and differentiation capabilities as BM-MSCs in both in vitro studies and in vivo by ex vivo transplantation into immuno-compromised mice. Experimentation of DPSC mirrored the previously established biological pathways of BM-MSCs. Odontoblasts are responsible for the formation of dentin and are suspected to arise from the dental pulp, but little information is known about the specific cell population that gives rise to the odontoblasts in the dental pulp cavity [6] and what biological scaffold is most effective in aiding in the production of odontoblasts.
Gronthos et al. [6] obtained pulp tissue from third molars of individuals aged 19-29. In vitro experimentation on differentiation potential of DPSC showed no formation of adipocytes, while BM-MSCs showed formation of adipocytes. Both cell populations were found to be clonogenic during in vitro manipulation. The DPSC were found to have a high proliferation capacity and proliferated more than the BM-MSCs [6]. BM-MSCs display bone formation on a hydroxyapatite/tricalcium phosphate (HA-TCP) powder, thus DPSC were observed in conjunction with HA-TCP powder to investigate if the DPSC would display a similar response as the BM-MSCs [6]. In vivo transplantation of DPSC resulted in odontoblast-like cells of human origin and contained the dentin-specific protein necessary to generate dentin [6]. This suggests the HA-TCP powder was conducive to the DPSC like the BM-MSCs.
Gronthos et al. [6] concluded that the DPSC are able to be considered a source of stem cells because of their profound regeneration, differentiation, and proliferation capabilities. DPSC may be harvested from one pulp cavity and applied to dentin regeneration in many teeth [6]. Granthos et al. [6] call for future research to investigate the biological scaffold that will amount to the most dentin regeneration associated with in vivo transplantation of DPSC.
Stem Cells from Human Exfoliated Deciduous Teeth
In 2003 Miura et al. [7] identified human exfoliated deciduous teeth (SHED) as a profound source of MSCs to be considered for use in autologous regenerative therapies. The cells were researched using prior methodology of umbilical cord derived stem cells and investigated specifically regarding the prior knowledge and research pertaining to umbilical cord derived stem cells. The study focused on ex vivo success through in vivo transplantation. An advantage of SHED as a source of stem cells is that they are easily obtained from an individual at a relatively young age. SHED are found in the dental pulp that is left in deciduous teeth that are lost and replaced with adult permanent teeth during adolescence in an individual’s lifetime. This makes SHED an ideal candidate for preservation through stem cell banking. The cells must be able to be preserved for use at a later time in the individual’s life if they are to be considered a viable source of stem cells for regenerative medicine and treatment. Similar to DPSC, SHED provide a relatively large source of stem cells as cells harvested from one pulp cavity may be also used in regenerative treatment for many other teeth of the individual.
Miura et al. [7] obtained pulp tissue from human exfoliated deciduous incisors from 7-8 year old individuals. The researchers suggest SHED may be originating from a perivascular environment [7] due to their origin and association with vasculature in the dental pulp. The SHED were investigated in both in vitro studies and in vivo by ex vivo transplantation into tissues of immunocompromised mice. Single cells were isolated and allowed to proliferate to form populations of cells as a way to measure their characteristic clonogenicity. The study also showed that the SHED have greater in vitro proliferation capabilities regarding formation of cell populations as compared to both BM-MSCs and DPSC [7]. The cell populations were then induced to differentiate and were successful in differentiating into the following cell types in vitro: odontoblast-like cells, adipocytes, and neuronal cells [7]. Furthermore, the cells differentiated into odontoblast-like cells on HA-TCP powder and were able to survive after in vivo transplantation.
The same MSCs markers as BM-MSCs and DPSC have displayed were observed on SHED suggesting similar differentiation capabilities among the various sources of stem cells. However, SHED was found to differentiate into odontoblasts in vivo but was not successful in differentiating into dentin pulp like complex like DPSC [7]. This suggests SHED possessing alternative differentiation capabilities or means of differentiating than those understood to be true of DPSC. Additionally, while the SHED were successful in producing odontoblast-like cells, SHED was unable to differentiate to produce osteoblasts. The cells were, however, able to induce varying degrees of bone formation in nearby host cells in vivo after transplantation. Miura et al. [7] proposed the SHED may play a role in bone formation around permanent adult teeth as the deciduous teeth are exfoliated.
Miura et al. [7] concluded that SHED are in fact a viable source of multipotent stem cells and have great potential in playing a role in regenerative medicine. The researchers suggest the differences between SHED and DPSC may be attributed to the developmental differences between adolescent deciduous teeth that are meant to be exfoliated and the development of permanent adult teeth that are made to survive an individual’s lifetime. Miura et al. [7] call for future research to investigate the purpose of SHED in adolescent deciduous pulp cavities, as understanding their purpose may lead to greater understanding of SHED and thus greater application of SHED in regenerative medicine.
Stem Cells from Periodontal Ligament of Periodontium
The periodontal ligament (PDL) was found by Seo et al. [8] to be a source of multipotent stem cells capable of playing a role in regenerating periodontal tissue. The cells of the PDL were investigated based on the known differentiation properties of the PDL, and this information was then used to obtain evidence that the PDL obtains stem cells and may be used for generation of cementum and new PDL specifically in vivo. Additionally, cellular markers and other multipotent determining properties of periodontal ligament stem cells (PDLSC) were compared to those of both DPSC and BM MSC. There exist other populations of cells that are capable of regeneration and possess many osteoblast-like properties, but no prior evidence of a stem cell population had been observed in the PDL [8].
Seo, et al. [8] extracted third molars from 19 to 29 year old humans harvesting the cells from the PDL were isolated and induced to proliferate in vitro. Interestingly, PDLSC were found to accumulate less calcium than DPSC [8]. Through in vitro studies, PDLSC were determined to have adipogenesis capabilities as well as proliferate into cementoblast and odontoblast-like cells. The ex vivo colonies were transplanted in vivo to immunocompromised mice through in vivo transplantation, specifically to the periodontal area of the mice. PDLSC developed cementum-like tissue different from tissues generated from BM MSCs and DPSC suggesting a difference in differentiation capabilities of the three cell groups [8]. The PDLSC; however, possess the same cellular markers expressed by BM-MSCs and DPSC that suggest a potential perivascular origin [8]. The PDLSC were also able to interact physiologically with the host collagen fibers suggesting they possess the capability to differentiate into cells to produce collagen fibers as well [8]. The in vivo integration of PDLSC into the periodontal area of which they were transplanted suggests great potential for future application of periodontal tissue regeneration using PDLSC despite their failure to attach to the bone of the teeth [8].
Seo,et al. [8] concluded that PDL is a viable source for obtaining multipotent mesenchymal stem cells and are consistent with the clonogenic, proliferative, and differentiating characteristic of mesenchymal stem cells from oral sources [8]. This was also confirmed by Kawanabe et al. [27] and Menicanin et al. [28] who confirmed the stem cell properties of PDLSC and further evaluated the post-transplant self-renewal potential of PDLSC. Menicanin et al. [28] further concluded that PDLSC possess significant capability to self-renew and regenerate periodontal tissue. Onizuke et al. [29] confirmed PDL as a source of stem cells and further analyzed the application of cell sheet engineer technologies in forming bioresorbable scaffolds to best support integration of the PDLSC for clinical applications. Seo et al. [8] call for future investigation of the cellular markers specific to PDLSC different from those expressed by BM MSCs and DPSC. Additionally they conclude future research should analyze PDLSC and their PDL tissue regeneration on a larger quantity of test animals and a larger size test subject population [8].
Stem Cells from Dental Follicle of Third Molars
Stem cells obtained from the dental follicle of third molars have also been determined to be a source of oral derived MSCs valuable in contributing to regenerative medicine [9]. The dental follicle was investigated based on its supposed capacity to differentiate into cementoblasts, osteoblasts, and periodontal ligament cells as well as its ease in accessibility from extracted teeth. Dental follicle cells possess the progenitors for osteoblasts that are responsible for aiding the development of many tooth related structures during growth and eruption of teeth and development of alveolar bone [9]. Findings from Gronthos et al. [5] research of DPSCs were applied to the study of DFSC to identify if DFSMs possess similar proliferation and differentiation capabilities.
Morsczeck et al. [9] studied human dental follicle cells of extracted third molars. The cells were isolated and induced to differentiate in both in vitro experimentation and ex vivo through in vivo transplantation using immunocompromised mice. A HA-TCP powder was included to induce uptake of the cells into the mice. It was found that DFSC behave similarly to the cells of the periodontium and are capable of regenerating cells types associated with the periodontium including alveolar bone, cementum, and periodontal ligament [9]. Morsczeck et al. [9] concluded that DFMSC can play a role in bone tissue engineering and possess the necessary cellular markers to be considered stem cells.
A similar study was conducted by Park et al. [30] that focused on comparing human skin derived MSC, BM-MSCs, and DFSC. Findings confirmed that DFSC have the capacity to differentiate into osteocytes, chondrocytes, and adipocytes in vitro [30]. Successful in vivo experimentation suggested that although the DFSC investigated possess MSC-like cellular markers similar to those of DPSC and BM-MSCs, further research should be conducted regarding the multipotency of the cells [30]. Osteogenic potential was however observed in both in vitro and in vivo cell populations of DFSC [30].
Tooth Germ Progenitor Cells from Third Molars
In 2007 Ikeda et al. [10] identified stem cells found in the tooth germ of developing third molars and termed them tooth germ progenitor cells. The tooth germ includes many tooth-related tissues in the oral cavity, but more specifically refers to the developmental stage of these tissues [31]. The purpose of the study was to research the morphology, proliferation, and multipotency of the TGPC, which were studied based on their ability to turn into hepatocytes mirroring the differentiation capabilities of BM-MSCs [32]; but different from previously discussed orally-derived MSCs. The researchers focused on investigating TGPC specifically regarding their use in treatment of liver diseases with the goal of restoring liver function caused by liver disease through the use of stem cells as an alternative to drug therapy [10]. TGPC obtained at a young age from the tooth germ of developing third molars are associated with few ethical concerns due to their routine extraction that normally results in disposal making them a great source for potential harvesting of stem cells.
The cell populations were tested both in vitro and ex vivo through in vivo transplantation into immunocompromised mice with HA-TCP use in transplantation [10]. In vitro the cells were found to maintain their morphology and clonogenic properties through the isolation and cell population formation and showed the ability to differentiate into cells of three germ layers [10]. The cells possessed the cellular markers specific to mesenchymal cells, and transcription factors were observed suggesting pluripotency and confirming their stem cell characteristics [10]. The mesenchymal character of these cells was also confirmed by Yalvac et al. [31] in 2010 as well as their ability to differentiate into progenitor cells with adipocyte, neural, and osteogenic characteristics. Yalvac and team also observed that TGPC expressed the cellular markers indicating stem cell character at a significantly higher level compared to DPSC [31]. In vitro cells displayed characteristics of osteoblasts, differentiated into neuronal cells and hepatocyte-like cells sharing characteristics of functional hepatocytes [10]. The Ikeda et al. [10] were the first to show that the neural crest derived cells can differentiate into cells from the endoderm lineage. After transplantation however, the in vivo cells showed great proliferation and indicated bone formation through the presence of osteocytes instead of only osteoblasts [10].
Ikeda et al. [10] concluded that TGPC are able to play an important role in regenerative medicine specifically related to the development of hepatocytes. Successful cloning and proliferation in both in vivo and in vitro studies confirmed the stem cell qualities of TGPC on treatment of liver disease. The team calls for future research to be conducted investigating the capacity to which the application of TGPC differentiating into hepatocytes may be applied to regenerative medicine regarding other tissues, including tissues of the oral cavity.
Gingiva Derived Mesenchymal Stem Cells
In 2009, Zhang et al. [11] identified human gingival tissue as a source of MSCs to be considered for tissue regeneration and treatment. The GMSC were investigated based on their abundance, accessibility, and tendency to be discarded as waste from surgical procedures. The focus of the study was to determine to what extent the GMSC are able to regenerate tissue and display associated anti-inflammatory characteristics, specifically in treatment of colitis [11].
The study was conducted using both in vitro and in vivo by ex vivo transplantation of human gingiva cells collected from the periodontium. The cells were isolated then showed higher proliferation capacity compared to BM-MSCs [11]. In vitro the GMSC displayed successful differentiation into adipocytes and osteoblasts of the mesoderm lineage as well as endodermal and neural ectodermal cells suggesting stem cell character [11]. Additionally the GMSC were shown to possess the cellular markers specific to stem cells and phenotype associated with mesenchymal progenitor cells further confirming their stem cell character [11].
The GMSC were transplanted using a HA-TCP carrier to mice infected with experimentally induced colitis. In vivo the cells were found to reduce disease severity and significantly reverse inflammation in the mice. Interestingly, the cells formed connective-like tissue instead of the expected osteoblast formation suggesting these cells may be useful in tissue regeneration [11]. Zhang et al. [11] call for future research to investigate if osteogenesis may be induced in vivo with the aid of a different transplant carrier.
More recent research has shown GMSC ability to differentiate into osteogenic cells in vivo [33]. Yang et al. [33] compared the capacity of both GMSC and PDLSC to periodontal disease treatment. GMSC exhibit enhanced ability upon transplantation to combat inflammation compared to PDLSC regarding in vivo osteogenesis which is explained by the unique characteristics associated with the gingival tissue regarding its immunoregulatory function and expedited healing [33]. Yang et al. [33] call for future research on determining the best feasible methods to incorporate the use of GMSC in therapeutic treatment of periodontal disease in tissue repair and regeneration in a clinical setting. Tomar et al. [34] compared GMSC and BM-MSCs specifically regarding if they may be considered a viable and sustainable source of stem cells to be used in regenerative medicine in a clinical setting. GMSC compared to BM-MSCs were found to provide a more stable source of stem cells regarding ability to proliferate and retain morphology in a long-term culture setting as is necessary for clinical application [34]. This was also confirmed by Sun et al. [35] and Tomasello et al. [36] who agree GMSC should be considered as a source for generation of bone in regenerative medicine.
Continued research on GMSC examine the advantages the cells offer to cutaneous wound healing [37], tendon regeneration[38], as well as regeneration of peripheral nerve defects in vivo [39]. The wide array of applications and characteristics these stem cells possess deem them potentially useful in tissue regeneration and treatment specifically in the oral region and in a clinical setting.
Alveolar Bone Derived Mesenchymal Stem Cells
In 2005 Matsubara et al. [12] identified the alveolar bone of the jaw as a viable source for stem cells to be used in autologous bone regeneration. An advantage of ABMSC as a source of stem cells is that they are easily obtained from an individual during dental implant surgery or extraction of third molars as the alveolar bone is often exposed or accessible during both of these surgical procedures. ABMSC are often less painful to obtain compared to BM-MSCs from other bones in the body [12].
Matsubara et al. [12] harvested human alveolar bone during normal third molar extractions. The cells were researched using prior methodology of BM-MSCs and investigated specifically regarding the prior knowledge and research pertaining to BM-MSCs. Single cells were isolated and allowed to proliferate to form populations of cells as a way to measure their characteristic clonogenicity. The study focused on ex vivo success through in vivo transplantation into immunocompromised mice. The researchers compared the ABMSC to BM-MSCs from the iliac bone regarding their proliferation and differentiation capacities [12]. Upon examination in vitro, ABMSC were found to possess similar stem cell character and cellular markers as BM-MSCs regarding osteogenic proliferation, but displayed significantly less adipocyte and chondrocyte potential compared to BM-MSCs [12]. Furthermore, the cells differentiated into odontoblast-like cells on a calcium phosphate scaffold and were able to survive after in vivo transplantation. Additionally, an association between age of patient and proliferation was observed showing a decline in proliferation with increased age of individual from which the cells were obtained. This decline was noticeably larger than that of the MSC from the iliac bone being studied. Matsubara et al. [12] acknowledge the varying research regarding this association and suggest banking of the stem cells obtained from extracted third molars of individuals at a relatively young age suggests the greatest potential for application in a clinical setting.
Matsubara et al. [12] concluded that ABMSC are in fact a viable source of multipotent stem cells and have great potential in playing a role in regenerative medicine, specifically regarding bone regeneration. They call for future investigation to determine if a different transport medium might increase the potential of ABMSC to differentiate into adipocytes and chondrocytes. Because of the strong osteogenesis potential of ABMSC, they are interested in determining the best clinical application of ABMSC in repairing bone in treatment of periodontal disease [12].
Another in vivo study focused on using stem cell sheets made from ABMSC as a non-scaffold approach for bone regeneration in the calvaria bone of rabbits. Liu et al. [40] observed greater osteogenic differentiation in ABMSC than in long bone MSC showing greater mineral deposition and cellular markers indicating osteogenesis. However, similar research by Pettersson et al. [41] showed that ABMSC and BM MSC display no significant difference in their ability to differentiate into osteogenic cells. Research is unclear regarding comparing the differentiation ability of ABMSC and BM-MSCs; however research concludes that ABMSC are a potential source of MSCs to be considered for regenerative treatment in craniofacial defects in a clinical setting [40-42].
Stem Cells from Apical Papilla
In 2006 Sonoyama et al. [15] identified apical papilla from the root of human teeth as a novel source of mesenchymal stem cells to be considered for oral tissue regeneration. The goal of the study was twofold: to confirm tooth apical papilla as a viable source of stem cells and to explore the combination of SCAP and PDLSC in regeneration of a root periodontal structure and associated tissues necessary to support an artificial crown [15]. A healthy and stable root is necessary to recover and maintain a functional tooth, and SCAP are thought to aid in regeneration of roots and surrounding tissues of teeth. SCAP provide a unique source of stem cells because they may be harvested from one source of apical papilla and applied to tissue regeneration in many surrounding teeth due to their great proliferation [15].
SCAP were collected and isolated from human apical papilla from normal extractions of third molars and allowed to proliferate to form cell populations for in vitro studies. The cells displayed cellular markers indicative of MSC character and were induced to successfully differentiate into osteoblasts and adipocytes [15]. Cells were then transplanted into immunocompromised mice with a HA-TCP carrier for further in vivo testing. The cells were able to generate both dentin and connective tissue in the in vivo model suggesting SCAP are a unique group of stem cells with different differentiation capacity than most dental derived stem cells [15]. In fact, SCAP showed greater differentiation into dentin than was expressed by DPSC [15].
An additional in vivo study was conducted using SCAP and PDLSC from swine followed by transplantation into immunocompromised mice. There exist similarities between human dental tissues and those found in swine thus conclusion can be made regarding human regenerative medicine based on the success of the tissue from swine [15]. Sonoyama et al. [15] used SCAP and PSLSC from the swine and observed formation of cementum on the scaffold. Formation of both dentin and PDL are necessary to regenerate a viable root periodontal structure capable of supporting a tooth.
Sonoyama et al. [15] propose that this is a viable method for functional restoration of teeth and supporting structures in a clinical setting. They call for future research to be conducted regarding the immunogenicity of the cells and suggest that SCAP may be an ideal candidate for stem cell banking [15]. Current research focuses on identifying the precise biomaterials that make up the environment in which SCAP best contributes to tissue regeneration such as oxygen level and ideal makeup of a transplant carrier [43-45].
Discussion of Stem Cell Banking
Stem cell banking may be loosely defined as the collection, isolation, and preservation of stem cells in preparation for use in regenerative treatment and therapy. The instance in which the cells are available for harvest might not align with when the cells are needed thus the cells must be stored and preserved in a manner that will maintain the functionality of the cells over a potentially long period of time. The process is unique because it provides the means for individualized autologous medicine that can be implemented throughout an individual’s lifetime [17]. Personalized advancements in medicine have the goal of increasing tolerance of the immune system and low rejection by host due to the host and the donor being the same individual. Specifically, dental stem cell banking utilizes the stem cells obtained from the oral region, and dental professionals, especially oral surgeons, are responsible for the collection of the cells. Dental stem cell banks were created in response to the identification of the oral region as a source of stem cells and current research investigates the best means of collecting, isolating, and preserving the cells so that the full potential of use of these cells may be reached in the advancement of personalized medicine.
The various oral sources of stem cells, as previously discussed, can be harvested during tooth extractions, the naturally occurring exfoliation of deciduous teeth, or through other minimally invasive surgeries. An advantage of utilizing orally derived stem cells is that there are fewer ethical concerns associated with their harvesting than with obtaining stem cells from embryological sources [17]. Additionally, the harvesting of DMSC is often simple and painless through a less invasive manner than stem cells harvested from other regions of the body as they are often a byproduct of a surgical procedure and would otherwise be discarded as waste. Dental stem cell banking, specifically using SHED, was found to be more cost efficient than banking stem cells obtained from cord blood [46]. If stem cells from cord blood were not obtained, stem cells from oral sources offer more opportunities in an individual’s life to harvest and bank stem cells [46]. Stem cell banking is a proactive form of personalized medicine as the individual chooses to bank their cells in preparation for their future medical needs. Current research focuses on determining the specific conditions for optimal collection, isolation, and preservation of dental stem cells. By identifying the specific condition in which to harvest and store the cells, the proliferation and differentiation capacities of the stem cells can be maintained.
Collection
Stem cell banking begins in the dental chair with the collection of the cells during a scheduled extraction or routine eruption of the tooth. The dental professional assisting in the process works with the stem cell bank to decide regarding the specificities of harvesting the cell and initial processing steps. Stem cell banks currently exist in Japan, the United States, India, and Norway. Stem cell bank information of FDA accredited banks in the United States is presented below:
- BioEDEN, USA (https://www.bioeden.com/us/)
- StemSave, USA (https://www.stemsave.com/)
- Store-a-Tooth by Provia Laboratories, LLC, USA (http://www.store-a-tooth.com/)
- National Dental Pulp Library, LLC (https://ndpl.net/)
It is important that the tooth from which the cells are collected is a normal healthy tooth with little to no decay due to pulpitis. Tsai et al. [47] investigated the impact of dental pulpitis on the effectiveness of stem cell banking and found it to be ineffective overall. A slower colonization process and increased pro-inflammatory cytokines were observed on the cells infected with pulpitis making then an un-ideal candidate for stem cell banking [47].
The cells are immediately placed in a container of sterile saline solution to maintain the vitality of the cells and to keep them from drying out during transport. The time between when the cells are collected and when the cells are processed is an important factor to determine the usefulness of the cells as the tissue may begin to degrade [48]. Perry et al. [49] studied the impact of time between collection and processing and was able to obtain cells for culturing up to 120 hours after extraction. This suggests that immediate processing post extraction is not a crucial factor necessary to effectively bank stem cells.
Perry et al. [49] also investigated the most effective collection transport medium to be used in the transition from the dental office to the lab where the cells are isolated. No notable difference was found between using PBS compared to HTS. PBS is the cheapest and most accessible medium to store in a dental office; however, there exist other transport media that can be considered for use as well. Slight alterations in the numbers of cells that are obtained for processing from the different medias are negligible because as the cells are processed, enough cells will be proliferated in the growing cell cultures [49].
Isolation
Isolation refers to the processing of the cells in preparation for preservation by the stem cell bank that is preserving them. As previously mentioned, isolation of cells is possible up to 5 days after the tooth containing the cells is extracted or removed from its source [49]. The pulp from the harvested tooth is disinfected, and the cells are isolated and cultured in MSC media. The focus of current research is identifying the optimal conditions in which the cells are allowed to proliferate and colonize. Factors to be taken into consideration are the process through which the cells are isolated, the extent to which the cells are digested, the means of cellular attachment during culturing, and the media, serum, and supplements that are used to enhance cellular growth and function during the culturing process. Perry et al. [49] identified proper sterilization of the harvested teeth as the most important aspect of forming uninfected and sterile cell cultures. Additionally, they investigated the efficiency of the cells establishing cell cultures after being isolated from the harvested teeth. Cell colonies were present and displayed viable cells after 14 days of isolation [49]. Throughout the study, the stem cell populations were tested to confirm their MSC character. The cells possessed the necessary cellular markers characteristic of MSC as well as displayed the ability to differentiate into osteogenic, adipogenic, and chondrogenic cells as is characteristic of MSC [49].
Preservation Cryopreservation and Magnetic Field Programmed Freezing
Cryopreservation is the process of cooling cells to an eventual temperature below 150°C by liquid nitrogen vapor. The cells must freeze carefully and quickly as to not form ice crystals which would result in cell death [50]. This is the main cellular damage that a cell may experience as a result of cryopreservation as well as mechanical stress from ice formed outside of the cell [51]. A cryoprotective agent (CPA) can be used in the vitrification process to maintain cell functionality during preservation, but the cryoprotectants are often harmful to the cells or even can cause cell death [52]. Through cryopreservation, cells are able to maintain their cellular markers, gene expression, and differentiation potential [49]. Perry et al. [49] determined this through the comparison of DPSC before and after cryopreservation. However, some changes in proliferation capacity are observed before and after cryopreservation [53-55].
In 2010 Kaku et al. [51] from Hiroshima University developed an alternative method for cryopreservation using a magnetic field programmed freezer instead of the established cryopreservation method. The novel method of preservation was created to combat both ice formations inside and outside of the cell as well as the toxicity associated with necessary levels of CPA [51]. This system of preservation is referred to as the cells alive system (CAS) and was adapted from an existing freezing methodology implemented in the food industry [51]. The CAS applies a weak magnetic field to the sample which lowers the freezing point. Kaku et al. [51] were able to determine the optimal hold time and plunging temperature necessary to freeze the cells without inducing obstruction to the cellular membrane Proliferation as well as differentiation capacity were observed for the cells to ensure they maintained their MSC-like character throughout the study [51]. Kaku et al. [51] specifically investigated the magnetic field freezing using PDLSC and found that they could proliferate equally as the teeth that were observed immediately post extraction. Another study by Lee et al. [56] observed similar results upon investigation of DPSC compared to cells from teeth that were not preserved [56].
Interestingly, viable stem cells have not only been obtained from post preservation isolated cell populations but also from teeth that were preserved intact [49,55,57]. This confirms that immediate processing post extraction is not a crucial factor necessary to effectively bank stem cells which would in turn result in lowered costs associated with banking stem cells as the processing of the cells is the most costly part of the process [48,49,53].
Conclusion
With the discovery and research of dental stem cells come great opportunities to utilize these cells in regenerative medicine and dental tissue repair. The aspects of each source of stem cells must be analyzed so that the best candidate for stem cell banking and the specific regenerative treatment may be used in personalized medicine. The eight sources of stem cells and their ability to successfully aid in regeneration of tissues have been analyzed: human exfoliated deciduous teeth, dental pulp stem cells, periodontal ligament stem cells, dental follicle stem cells, tooth germ progenitor cells, gingival-derived mesenchymal stem cells, alveolar bone mesenchymal stem cells, and stem cells from the apical papilla. The relatively recent developments in stem cell biology make banking dental stem cells a feasible option for regeneration of tissues and other oral structures. As with any new developments in research, however, there exist certain limitations associated with achieving a consistent application in a clinical setting. Despite the many advancements that have been made in the field, the cellular conditions in which the stem cells may be collected, isolated, and preserved must be improved and perfected before clinical application is regularly implemented. The viability and stability of the preserved stem cells must be considered further so that the preservation efforts do not result in loss. Appropriate long term double-blind randomized clinical trials are still necessary before stem cell therapy may become a normalcy in a clinical setting. Additionally, current stem cell research is limited to animal models and must be applied to human models before clinical application may occur. The opportunity for immune rejection exists, but as is in the case with stem cells, immune rejection is limited due to the autologous nature of the cells. With continued research and developments in the field of stem cell biology, the dental stem cells support the continued development and betterment of the stem cell banking industry.
References
- Paz AG, Maghaireh H, Mangano FG. (2018) Stem Cells in Dentistry: Types of Intra- and Extraoral Tissue-Derived Stem Cells and Clinical Applications. Stem Cells Int. 2018.
- Caplan AI. (1991) Mesenchymal stem cells. J Orthop Res. 9(5):641-50.
- Mao JJ. (2008) Stem cells and the future of dental care. N Y State Dent J. 74(2):20-4.
- Ohkoshi S, Hirono H, Nakahara T, Ishikawa H. (2018) Dental pulp cell bank as a possible future source of individual hepatocytes. World J Hepatol. 10(10):702-7.
- Gronthos S, Brahim J, Li W, Fisher LW, Cherman N, Boyde A, et al. (2002) Stem cell properties of human dental pulp stem cells. J Dent Res. 81(8):531-5.
- Gronthos S, Mankani M, Brahim J, Robey PG, Shi S. (2000) Postnatal human dental pulp stem cells (DPSCs) in vitro and in vivo. Proc Natl Acad Sci USA. 97(25):13625-30.
- Miura M, Gronthos S, Zhao M, Lu B, Fisher LW, et al. (2003) SHED: stem cells from human exfoliated deciduous teeth. Proc Natl Acad Sci USA. 100(10):5807-12.
- Seo B, Miura M, Gronthos S, Bartold PM, Batouli S, et al. (2004) Investigation of multipotent postnatal stem cells from human periodontal ligament. Lancet. 364(9429):149-55.
- Morsczeck C, Götz W, Schierholz J, Zeilhofer F, Kühn U, Möhl C, et al. (2005) Isolation of precursor cells (PCs) from human dental follicle of wisdom teeth. Matrix Biol. 24(2):155-65.
- Ikeda E, Yagi K, Kojima M, Yagyuu T, Ohshima A, et al. (2008) Multipotent cells from the human third molar: feasibility of cell-based therapy for liver disease. Differentiation. 76(5):495-505.
- Zhang Q, Shi S, Liu Y, Uyanne J, Shi Y, Shi S, et al. (2009) Mesenchymal stem cells derived from human gingiva are capable of immunomodulatory functions and ameliorate inflammation-related tissue destruction in experimental colitis. J Immunol. 183(12):7787-98.
- Matsubara T, Suardita K, Ishii M, Sugiyama M, Igarashi A, et al. (2005) Alveolar bone marrow as a cell source for regenerative medicine: differences between alveolar and iliac bone marrow stromal cells. J Bone Miner Res. 20(3):399-409.
- Rai S, Kaur M, Kaur S. (2013) Applications of Stem Cells in Interdisciplinary Dentistry and Beyond: An Overview. Ann Med Health Sci Res. 3(2):245-54.
- Tamaki Y, Nakahara T, Ishikawa H, Sato S. (2013) In vitro analysis of mesenchymal stem cells derived from human teeth and bone marrow. Odontology. 101(2):121-32.
- Sonoyama W, Liu Y, Fang D, Yamaza T, Seo B, et al. (2006) Mesenchymal stem cell-mediated functional tooth regeneration in swine. PLoS ONE. 1:e79.
- Bluteau G, Luder HU, De Bari C, Mitsiadis TA. (2008) Stem cells for tooth engineering. Eur Cell Mater. 16:1-9.
- Arora V, Arora P, Munshi AK. (2009) Banking stem cells from human exfoliated deciduous teeth (SHED): saving for the future. J Clin Pediatr Dent. 33(4):289-94.
- Ullah I, Subbarao RB, Rho GJ. (2015) Human mesenchymal stem cells-current trends and future prospective. Biosci Rep. 35(2).
- Wei X, Yang X, Han ZP, Qu FF, Shao L, et al. (2013) Mesenchymal stem cell: a new trend for cell therapy. Acta Pharmacol Sin. 34(6):747-54.
- Bianco P, Robey PG, Simmons PJ. (2008) Mesenchymal Stem Cells: Revisiting History, Concepts, and Assays. Cell Stem Cell. 2(4):313-9.
- Imamura H, Adachi T, Kin T, Ono S, Sakai Y, et al. (2018) An engineered cell sheet composed of human islets and human fibroblast, bone marrow-derived mesenchymal stem cells, or adipose-derived mesenchymal stem cells: An in vitro comparison study. Islets. 10(3):e1445948.
- Mutlu L, Hufnagel D, Taylor HS. (2015) The endometrium as a source of mesenchymal stem cells for regenerative medicine. Biol Reprod. 92(6):138.
- Ding D, Chang Y, Shyu W, Lin S. (2015) Human umbilical cord mesenchymal stem cells: a new era for stem cell therapy. Cell Transplant. 24(3):339-47.
- Harvanova D, Tothova T, Sarissky M, Amrichova J, Rosocha J. (2011) Isolation and characterization of synovial mesenchymal stem cells. Folia Biol (Praha). 57(3):119-24.
- Ab Kadir R, Zainal Ariffin SH, Megat Abdul Wahab R, Kermani S, Senafi S. (2012) Characterization of mononucleated human peripheral blood cells. Sci World J. 2012:843843.
- Pierdomenico L, Bonsi L, Calvitti M, Rondelli D, Arpinati M, et al. (2005) Multipotent mesenchymal stem cells with immunosuppressive activity can be easily isolated from dental pulp. Transplantation 80(6):836-42.
- Kawanabe N, Murata S, Murakami K, Ishihara Y, Hayano S, et al. (2010) Isolation of multipotent stem cells in human periodontal ligament using stage-specific embryonic antigen-4. Differentiation. 79(2):74-83.
- Menicanin D, Mrozik KM, Wada N, Marino V, Shi S, et al. (2014) Periodontal-ligament-derived stem cells exhibit the capacity for long-term survival, self-renewal, and regeneration of multiple tissue types in vivo. Stem Cells Dev. 23(9):1001-11.
- Onizuka S, Iwata T. (2019) Application of Periodontal Ligament-Derived Multipotent Mesenchymal Stromal Cell Sheets for Periodontal Regeneration. Int J Mol Sci. 20(11).
- Park B, Kang E, Byun J, Son M, Kim H, et al. (2012) In vitro and in vivo osteogenesis of human mesenchymal stem cells derived from skin, bone marrow and dental follicle tissues. Differentiation. 83(5):249-59.
- Yalvac ME, Ramazanoglu M, Rizvanov AA, Sahin F, Bayrak OF, et al. (2010) Isolation and characterization of stem cells derived from human third molar tooth germs of young adults: implications in neo-vascularization, osteo-, adipo- and neurogenesis. Pharmacogenomics J. 10(2):105-13.
- Theise ND, Nimmakayalu M, Gardner R, Illei PB, Morgan G, et al. (2000) Liver from bone marrow in humans. Hepatol. 32(1):11-6.
- Yang H, Gao L, An Y, Hu C, Jin F, et al. (2013) Comparison of mesenchymal stem cells derived from gingival tissue and periodontal ligament in different incubation conditions. Biomaterials. 34(29):7033-47.
- Tomar GB, Srivastava RK, Gupta N, Barhanpurkar AP, Pote ST, et al. (2010) Human gingiva-derived mesenchymal stem cells are superior to bone marrow-derived mesenchymal stem cells for cell therapy in regenerative medicine. Biochem Biophys Res Commun. 393(3):377-83.
- Sun Q, Nakata H, Yamamoto M, Kasugai S, Kuroda S. (2019) Comparison of gingiva-derived and bone marrow mesenchymal stem cells for osteogenesis. J Cell Mol Med. 23(11):7592-601.
- Tomasello L, Mauceri R, Coppola A, Pitrone M, Pizzo G, et al. (2017) Mesenchymal stem cells derived from inflamed dental pulpal and gingival tissue: a potential application for bone formation. Stem Cell Res Ther. 8(1):179.
- Zhang Q, Su W, Shi S, Wilder-Smith P, Xiang AP, et al. (2010) Human gingiva-derived mesenchymal stem cells elicit polarization of m2 macrophages and enhance cutaneous wound healing. Stem Cells. 28(10):1856-68.
- Moshaverinia A, Xu X, Chen C, Ansari S, Zadeh HH, et al. (2014) Application of stem cells derived from the periodontal ligament or gingival tissue sources for tendon tissue regeneration. Biomaterials. 35(9):2642-50.
- Zhang Q, Nguyen PD, Shi S, Burrell JC, Cullen DK, et al. (2018) 3D bio-printed scaffold-free nerve constructs with human gingiva-derived mesenchymal stem cells promote rat facial nerve regeneration. Sci Rep. 8(1):6634.
- Liu Y, Wang H, Dou H, Tian B, Li L, et al. (2020) Bone regeneration capacities of alveolar bone mesenchymal stem cells sheet in rabbit calvarial bone defect. J Tissue Eng. 11:2041731420930379.
- Pettersson LF, Kingham PJ, Wiberg M, Kelk P. (2017) In Vitro Osteogenic Differentiation of Human Mesenchymal Stem Cells from Jawbone Compared with Dental Tissue. Tissue Eng Regen Med. 14(6):763-74.
- Mason S, Tarle SA, Osibin W, Kinfu Y, Kaigler D. (2014) Standardization and safety of alveolar bone-derived stem cell isolation. J Dent Res. 93(1):55-61.
- Remy M, Ferraro F, Le Salver P, Rey S, Genot E, et al. (2019) Isolation and Culture of Human Stem Cells from Apical Papilla under Low Oxygen Concentration Highlight Original Properties. Cells. 8(12).
- Ha M, Athirasala A, Tahayeri A, Menezes PP, Bertassoni LE. (2020) Micropatterned hydrogels and cell alignment enhance the odontogenic potential of stem cells from apical papilla in-vitro. Dent Mater. 36(1):88-96.
- Tatic N, Rose FR,, Felicity RAJ, des Rieux A, White LJ. (2019) Stem cells from the dental apical papilla in extracellular matrix hydrogels mitigate inflammation of microglial cells. Sci Rep. 9(1):14015.
- Popuri SK. (2018) Concerns of a Pediatric Dentist in Dental Stem Cells: An Overview. Open Dent J. 12:596-604.
- Tsai AI, Hong H, Lin W, Fu J, Chang C, et al. (2017) Isolation of Mesenchymal Stem Cells from Human Deciduous Teeth Pulp. Biomed Res Int. 2017:2851906.
- Woods EJ, Benson JD, Agca Y, Critser JK. (2004) Fundamental cryobiology of reproductive cells and tissues. Cryobiology. 48(2):146-56.
- Perry BC, Zhou D, Wu X, Yang F, Byers MA, et al. (2008) Collection, cryopreservation, and characterization of human dental pulp-derived mesenchymal stem cells for banking and clinical use. Tissue Eng Part C Methods. 14(2):149-56.
- Zhurova M, Woods EJ, Acker JP. (2010) Intracellular ice formation in confluent monolayers of human dental stem cells and membrane damage. Cryobiology. 61(1):133-41.
- Kaku M, Kamada H, Kawata T, Koseki H, Abedini S, et al. (2010) Cryopreservation of periodontal ligament cells with magnetic field for tooth banking. Cryobiology. 61(1):73-8.
- Fahy GM, Wowk B, Wu J, Phan J, Rasch C, et al. (2004) Cryopreservation of organs by vitrification: perspectives and recent advances. Cryobiology. 48(2):157-78.
- Woods EJ, Perry BC, Hockema JJ, Larson L, Zhou D, et al. (2009) Optimized cryopreservation method for human dental pulp-derived stem cells and their tissues of origin for banking and clinical use. Cryobiology. 59(2):150-7.
- Ma L, Makino Y, Yamaza H, Akiyama K, Hoshino Y, et al. (2012) Cryopreserved dental pulp tissues of exfoliated deciduous teeth is a feasible stem cell resource for regenerative medicine. PLoS ONE. 7(12):e51777.
- Lindemann D, Werle SB, Steffens D, Garcia-Godoy F, Pranke P, et al. (2014) Effects of cryopreservation on the characteristics of dental pulp stem cells of intact deciduous teeth. Arch Oral Biol. 59(9):970-6.
- Lee S, Chiang P, Tsai Y, Tsai S, Jeng J, et al. (2010) Effects of cryopreservation of intact teeth on the isolated dental pulp stem cells. J Endod. 36(8):1336-40.
- Gioventu S, Andriolo G, Bonino F, Frasca S, Lazzari L, et al. (2012) A novel method for banking dental pulp stem cells. Transfus Apher Sci. 47(2):199-206.