Potential Mechanism of Nanoparticles Targeting Cancer Metabolic Pathways: Novel Approaches for Cancer Treatment
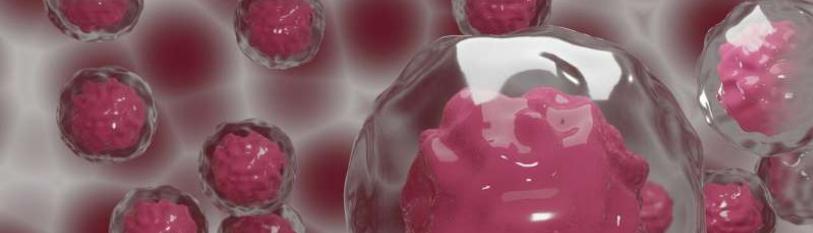
Zia Ud Din*, Yasmeen Khan, Amn Zia, Nasira Anbreen and Rifasa Sultan
Department of Biosciences COMSATS University Islamabad, Pakistan
*Corresponding author: Zia Ud Din, Department of Biosciences COMSATS University Islamabad, Pakistan
Citation: Din ZU, Khan Y, Zia A, Anbreen N, Sultan R. (2023) Potential Mechanism of Nanoparticles Targeting Cancer Metabolic Pathways: Novel Approaches for Cancer Treatment. Adv Clin Med Res. 4(3):1-24.
Received: August 16, 2023 | Published: September 09, 2023
Copyright© 2023 genesis pub by Din ZU, et al. CC BY-NC-ND 4.0 DEED. This is an open-access article distributed under the terms of the Creative Commons Attribution-Non Commercial-No Derivatives 4.0 International License., This allows others distribute, remix, tweak, and build upon the work, even commercially, as long as they credit the authors for the original creation.
DOI: https://doi.org/10.52793/ACMR.2023.4(3)-61
Abstract
Cancer is the most prevalent disease worldwide that has respected no borders. Despite of the intensive research in the field of medical, no satisfactory cancer treatment strategy has been developed. Conventional cancer therapies have been facing multiple challenging factors that have made cancer difficult to treat. Poor drug delivery system, anticancer drug resistance and metastasis are the prime limitations in the conventional cancer therapies, posing serious effects on the health of the cancer patients. Nanotechnology is an exciting new field of study that has proven its significance in multiple areas including medicines. It has also provided potential views for cancer treatment, enabling more effective, target-specific treatment regimens with fewer adverse effects. Multiple nanotechnology-based cancer treatments are being studied and analyzed for which numerous varieties of nanoparticles are used. The current review highlights the novel approaches and current practices of nanomaterial-based cancer treatment strategies targeting multiple cancer metabolisms. Furthermore, it will spotlight the advantages of NPs- based treatments over conventional methods, challenges and future perspectives of nanotechnology-based cancer therapies.
Keywords
Anticancer drugs; Nanoparticles; Drug delivery, Cancer metabolic pathways; Nanomedicines
Introduction
Cancer is the most prevalent disease worldwide, has taken more than 10 million lives in the year 2020 [1]. Cancer cells exhibit uncontrolled proliferation, transformation, and the capability to metastasize to distant organs, posing a threat to normal body cells [2]. However, to fulfill its energy requirement for its unregulated proliferation and migration, tumor cells acquired energy and raw material through unusual metabolic pathways [3]. The reason behind acquiring energy from unusual metabolic pathways is the more rigorous metabolism of cancer cells than normal cells [4]. In cancer cells, various signaling pathways that promote carcinogenesis collectively control three prime metabolic pathways [5]. However, lipids, glucose and protein metabolism are included in these metabolic pathways [6]. The distinct metabolism in tumor cells indicates that changes in the metabolic process are crucial for tumor development [7]. However, recent advancement in the area of medicine, conventional cancer treatments such as chemotherapy, have failed due to various factors connected with cancer. Normally tumor cells resist therapeutic drugs and other therapies such as chemotherapy [6]. The current chemotherapeutic drugs not only destroy the tumor cells while also kill normal body cells. Therefore, the administration of these toxic drugs can lead to severe side effects and may sometimes become the cause of patient’s death [8]. Some other limitations associated with free drug delivery system include reduced permeability and limited biocompatibility [9]. The greatest disadvantages of the free drug delivery are the nonspecific targeting of cancerous sites. Similarly, in radiotherapy there is no cell specificity that leads to similar side effects in the cancer patients. Drugs resistance is another challenge where sometimes the anticancer drugs stop performing their function completely [10]. Medical field are facing some other major challenges in the treatment of cancer like reoccurrence and metastasis of cancer cells. Some cancers cells escape the cancer treatment and reappear after being ready for metastasis [11]. They uncontrollably grow again and form a tumor. This phenomenon is also termed relapse [12]. Sometimes, the relapse occurs within days after treatment, whereas it can also take months or years to reoccur [13]. In metastasis, it becomes difficult to treat cancer when the tumor spreads in the adjacent tissues and even in the entire body [14]. Targeting cancer all over the body with drugs and other therapies becomes a challenge for doctors and patients [15]. For instance, healthy tissues are also affected due to chemotherapy, so it's life-threatening to expose the whole body to chemotherapy [16][17]. An overview of conventional methods of cancer treatments is summarized in (Figure 1). Keeping in view the challenges to cancer treatments, there is a dire need for treatments that are inexpensive, more precise, and have potential to overcome the challenges mentioned above [18]. now a days nanoparticles are considered a therapeutic strategies for cancer treatment [19]. It has grabbed the attention of cancer researchers due to its unique properties, which have been opening the gates to novel methods of cancer diagnosis, characterization, and treatment [20]. This review will highlight the novel approach of nanoparticles to treat cancer, overcoming the limitations of the conventional cancer treatment methods, and the study of underlying mechanisms of NPs to target cancer metabolic pathways.
Figure 1: Conventional Methods of treating cancer.
Application of Nanoparticles in Cancer Therapy
In medical field nanoparticles with size ranging between 1-100 nm are used [21] . These NPs are used in therapeutic drugs synthesis, designing devices and manufacturing [22]. NPs differ from conventional macromolecules due to their distinct optical, magnetic, and electrical characteristic that manifest at nanoscale [23]. Super magnetic behavior, High surface-to-volume ratio, improved electrical conductivity, and unique fluorescence properties are the typical characteristics of the nanoparticles [24]. In the field of medical, nanoparticles are being applied for drug transportation with controlled release [25]. Increased permeability and enhanced biocompatibility are also the prominent features of the nanoparticles [26]. These unique properties of nanoparticles make them potential for use in cancer therapeutics [27]. Nanoparticles has the potential to increase the specificity of the drugs in targeted therapy because of its high surface to volume ratio property, therefore improves the efficiency of the nanoparticles-based treatment although minimizing its toxicity to normal cells [28]. Photodynamic therapy (PDT) and Photo thermal therapy (PTT) are the two mechanism of treatment associated with optical interference [29]. Photodynamic treatment (PDT) produces cytotoxic reactive oxygen species as well as singlet oxygen., leading to cell death [30]. In PPT based treatment, materials with maximum photo thermal transforming properties are used that have the ability to elevate the temperature of the cancerous location ultimately leading to necrosis. These methods are considered advanced cancer treatment methods with impressive application and materials utilized for these therapies are under intensive investigation [30]. Some nanoparticles can also be used in PDT and PTT cancer treatment methods due to its distinct fluorescence properties [31]. The unique and distinct properties of nanoparticles enable them to be used for cancer diagnosis and treatment [32]. Because of their excellent targeting accuracy, small size, controlled release, and ability to avoid the immune response, super paramagnetic iron oxide particles (SPIONs) have the potential to treat hyperthermia [33]. To eliminate cancer, targeting tumorous sites is some conventional therapies [34]. It is now conceivable for these carriers to enter malignant locations and release therapeutic drugs by combining the enhanced permeability and retention effect (EPR) with active targeting techniques like as modified nanoparticles (NPs) or dendrimers [35]. In addition, biomaterials antibodies which aim at targeting overexpressed specific antigens found on cancer cell surfaces are extensively used [36]. After the process of endocytosis, encapsulated drugs are released and exert cytotoxic effect or nuclear materials triggers cell apoptosis, depends on the encapsulated drugs [37]. Progress has been achieved in the delivery of nucleic acid nanoparticle-based drug delivery systems (DDS) targeting transporters, utilizing platforms such as exosome, liposomes, dendrimers and polymeric nanoparticles are extensively explored and researched in cancer therapy [38]. Targeted cancer therapy aims at targeting specific cancer metabolic pathways and proteins involved in the cancer progression [39].
Advantages of NPs-based cancer treatment over conventional treatment methods
The prime advantage of the nanoparticles is the targeted administration directly to the metabolic pathways of cancer [40] . Intensive research and progress has been made for targeted drug delivery in cancers. It is accomplished by either active or passive targeting [41]. Active targeting comprises the attachment of nanoparticles to antibodies, peptides, aptamers, and other small molecules, whereas passive targeting involves better retention time and permeability [42]. Targeted nanoparticles-based drug delivery helps minimizing harms to healthy cells, prevent drug degradation, increases half-life, drug loading capacity and solubility [43]. NPs-based cancer treatment provides maintenance of better specificity, compatibility with biological systems, reduce toxicity, longer duration of effectiveness, regulated medication release, and better drug carrying capacity, overcoming the limitations in the conventional chemical methods of cancer treatment [44]. When drugs are encapsulated with NPs, anticancer therapies are less likely to be resistant to target cell resistance, i.e. the anticancer drug Metformin coupled with NPs is more effective than using Met alone to treat cancer [45].
Moreover, target-specific NPs can also be used to regulate the genes involved in the regulation of metastasis and have potential to inhibit them [46]. Intravesical delivery of mucoadhesives NPs along with Lysine Demthylase 6A (KDM6A-mRNA) could inhibit the metastasis of the bladder cancer [47]. Despite the intensive research for cancer therapies using nanoparticles, only a limited number of nano-drugs has been created successfully and are in clinical use. These nanoparticles can be typically classified into multiple categories shown in (Figure 2) [48].
Figure 2: A range of nanomaterials finds application in the field of cancer treatment. a Nanoparticles. b Solid lipid nanoparticles. c. Liposomes d. Nanostructured lipid carriers. e Nanoemulsions. f Graphene. g Dendrimers. h Metallic nanoparticles. PEG, poly (ethylene glycol) [48].
Metabolic Pathways Involved in the Progression of Cancer
Oncogenes activation and tumor suppressors’ loss have the key role in the promotion of reprogramming of the metabolism of cells in cancer [49]. This alteration leads to enhancement in the uptake of nutrients that are required to supply energy to pathways of biosynthesis [50]. Solid tumors experience lack of nutrients [51]. To overcome this limitation metabolic flexibility is adapted by the cancer cells that help in sustainability of growth and survival [52]. Adjustment of metabolic pathways is assumed as the one of the major hallmarks of the cancer [53]. Hence it has been the focused area of cancer research over the last decade. When nutrients are in abundance, more nutrients are exquisite by cancer signaling pathways to facilitate the assimilation of carbon into various macromolecules including proteins, nucleic acids and lipids [54][55]. These cellular activities facilitate the growth and multiplication of cells [56]. In oncogenic pathways, glucose and glutamine metabolism are reprogrammed consistently by mutations [57]. The mutations are evident in Tumor Protein 53, Myc proteins, the Ras-assosiated oncogenes, along with the signaling pathways involving PI3 kinase (PI3K) and the upstream kinase liver kinase B1- Activated protein kinase (LKB1-AMP) kinase (AMPK), among others as shown in (Figure 3) [58,59]. Oncogenic Ras is implicated in the activation of both the glucose uptake via enhanced activity of Glucose transporter 1(GLUT1) and uptake of glucose uptake with the help of anabolic pathways [60]. Ras also has its key role in the regulation of glutamine metabolism where it directs glutamine carbon into such pathways that support cell survival and growth [61]. Enhanced MYC expression is involved in the exhibition of multiple metabolic effects [62] including enhanced glycolysis [63] enhanced glutamine catabolism [64], enhanced mitochondrial biogenesis and enhanced metabolism of glutamine, culminating in assimilation of biomass [65]. The convergence of multiple pathways on glutamine and glucose indicates an abundance of these nutrients, which then enter the central metabolism [66]. Glutamine also provides its two nitrogen atoms for the synthesis of nucleotides, hexosamines and amino acids essential for growth [67]. In a nutshell, studies cancer studies reveal that cancer cells bear much more complicated metabolic requirements and multiple pathways complement production of biomass dependent on glutamine and glucose [68]. Some of major pathways are discussed here to elaborate how they contribute to cell proliferation and growth.
Although the blood pressure inside the tumors is low, lymphatic deficiency and leaky blood vessels come into play and make the blood serum protein reachable to cancer cells [69]. Ras- driven tumors experience maximum number of macropinocytosis which facilitate them to uptake extracellular proteins (ECPs) Fig 2b [70]. It is also has been observed that in case of nutrient starvation by blocking of mTORC1 signaling pathway forces tumor cells exhibit dependency on extracellular macromolecules rather than amino acids [71][72]. In an experiment, Phosphatase and tensin homolog (PTEN-deficient prostate cancer cell lines and K-Ras driven pancreatic tumors were studied which revealed that AMPK activation and suppression of mTORC1 in a state of glucose and amino acid deprivation tumor proliferation is facilitated by clearing cellular debris [72]. Cell Biomass is developed facilitated by amino acids from cell debris [73]. Moreover, pancreatic ductal adenocarcinoma (PDAC) has the ability to incorporate collagen I and IV via glucose and glutamine starvation [73]. PDAC cells degrade the encapsulated collagens taken up by lysosomes for the provision of proline [74]. Proline enters the tricarboxylic acid cycle via extracellular signal-regulated kinase 1/2 pathways, which results in ATP generation and, ultimately, cell viability [75]. In vitro Studies reveals that albumin is the main source of nutrients for cancer cell survival [76]. An in vivo study exhibited that PDAC cells have the ability to ingest albumin and utilize amino acids generated from albumin in other metabolic pathways whereas this property does not belong to normal cells [77] Tumors cells can also utilize extracellular lipids for cell proliferation and survival in a state of hypoxia or nutrient limitation [78]. For the reproduction of membrane and proliferation cancer cells need extracellular lipids and fatty acids [79]. Non-essential fatty acids are produced by tumor cells in normal condition in which oxygen is readily available [80]. On the other hand, Ras-driven cancers or hypoxic depend on the scavenging fatty acids from tumor microenvironment [81]. Ras-driven cancer cells have the ability to internalize lipids with one fatty acid tail to fulfill the requirement of lipid [82]. Cancer cells have a decrease in lipid droplet content during periods of famine. Prostate cancer cells lacking PTEN rely on lipids derived from cellular waste [83]. Invasive breast tumor in co-culture with obese adipocytes, exhibit increased proliferation and migration [84]. When fatty acids from adipocytes are transferred to invasive breast cancer cells, it activates adipose triglyceride lipase (AGTL) - induced lipolysis and fatty acid oxidation in mitochondria [85].
Recent studies have revealed the role of acetate and enzymes involved in acetate metabolizing in cancer cells [86]. Infusion of 13C-glucose and 13C-acetate into mice with orthotropic glioblastomas exhibited that both substrates could be oxidized [87]. But, in the blood stream the tumors oxidized at higher rate than did the healthy brain tissues in the surrounding [88]. In metastatic brain tumors acetate oxidation was observed that proposed that this pathway is a general feature of tumor in the brain [89,90]. Also in gliomas and brain metastases extensive acetate oxidation was revealed when similar mixture of 13C-glucose and 13C-acetate was infused in the human patients [91,92]. To explore the importance of tumor cells prolifiration, a mouse model of Acyl-CoA Synthetase Short Chain 2 of deficiency was used [93,94]. These ACSS2-deficient embryonic fibroblasts are not able to utilize exogenous acetate for histone acetylation and lipogenesis [95]. Moreover, ACSS2 knockout in two models of hepatocellular carcinoma reduces the burden of tumor [93,96]. Selective ACSS2 inhibitors development proposed the idea that modulating acetate metabolism may lead to strong therapeutic approach in some forms of cancer [97-99]. Keeping in view the significance of above mentioned metabolic pathways of cancer, some key points of metabolism in these pathways can be targeted with the help of Nanoparticles to find out potential therapeutic approaches of cancer. In this review, application of NPs is explored in different forms of cancer with an objective to find out a successful treatment of cancer.
Application of JX06 Nanoparticles for Targeting Cancer Metabolism
Diabetes is an illness associated with the appearance of endometrial cancer (EC) incidence and its poor detection [100]. But for EC patients with diabetes, there is no effective treatment available [101]. In an experimental study, JX06 nanoparticles were applied for targeting cancer metabolism by inhibiting 3-Phosphoinositide-dependent kinase 1 in combination with Metformin for EC patients with diabetes disease. To find out novel therapeutic targets, Ishikawa cell lines were cultured with high glucose (IshikawaHG) [102,103]. The condition of IshikawaHG resembles much like hyperglycemia in EC cancer with diabetes (EC+/dia+) [104]. It exhibited glucose metabolic reprogramming in IshikawaHG as an increased glycolysis level and oxidative phosphorylation reduction was observed. With the development of IshikawaHG cell lines, exposure to high-glucose culture promoted the convergence of cancer metabolism to glycolysis of EC from oxidative phosphorylation. Moreover, the identified enzyme behind the promotion glycolysis of IshikawaHG was pyruvate dehydrogenase kinase 1 (PDK1). Furthermore, a novel PDK1 inhibitor, JX06 NPs, in combination with the diabetic drug Metformin (Met), greatly inhibits the IshikawaHG proliferation, although Met faces resistance in IshikawaHG cell lines. For encapsulation of JX06, a biodegradable polymer that is sensitive to reduction [105] was utilized to develop nanoparticles (JX06-NPs) for the delivery of the drug. The in vitro experiment revealed that JX06 Nanoparticles have a significant inhibitory effect on patient- derived EC cells (PDC) and the IshikawaHG cell lines than the simple use of JX06. In addition, it was also found that JX06-NPs have the capacity to accumulate in the tumor proliferation of mice having endometrial cancer with diabetes (miceEC+/dia+) as a result of intravenous injection. The results also showed that JX06-NPs in combination with Met have the power to significantly inhibit the tumor growth[106] of EC in mice with diabetes. The mass spectrometry- based on proteomic screening [107] revealed the 3.3 times higher expression of the key enzyme in glycolysis, PDK1. In the state of knockdown of PDK1 using shRNA, significant inhibition of proliferation, glycolysis, invasion, and AKT/GSK3𝛽/𝛽-catenin signaling pathway of IshikawaHG was observed. The schematic representation of downregulation of PDK1 is shown in (Figure 3) [108]. It confirmed that PDK1 could have a significant role in the malignancy of the EC cells activated by high glucose. Inhibition activity of JX06 [109] was 2.5 times greater than Met only on IshikawaHG cell lines. Also, the apoptotic rate was higher on Ishikawa HG cell lines induced by JX06 based nanoparticles with combined Met [110]. The mechanism of inhibition is purposed to be associated with inhibition of the glycol sis pathway and oxidative phosphorylation. Mouse models have been established that exhibited promising results. To sum up, the study revealed that JX06-NPs, when used in combination with Met, have the ability to target the plasticity of cancer metabolism, which resulted in the significant inhibition of the proliferation of endometrial cancer. Hence, it put forwards a novel adjuvant-based therapy for EC patients with diabetes. The experiment suggests that Met, in combination with JX06-NPs, exhibits anticancer effects, and adjuvant treatment for patients EC+/dia+ can give promising results for future clinical practices. By combining Met with JX06-NPs, outstanding anticancer effects can be achieved. Despite the successful results of in vitro trials, there are challenges in the application of JX06 NPs need to be addressed and resolved [110].
Figure 3: Schematic representation of JX06 nanoparticles downregulating the expression of PDK1 enzyme and (Met) to target plasticity of cancer as well as glucose metabolism reprogramming for harmonious anticancer effects. JX06 was encapsulated to create JX06 NPs with the help of reduction sensitive polymer (P1). These NPs were intravenously injected into mice while Met was given orally to mice. Met decrease the blood glucose level and stop mitochondrial complex I activity and oxidative phosphorylation. Therefore, JX06-NPs have the ability to excrete JX06 after injecting into cancer cells, and reducing the expression of PDK1, which have the potential to subsequently inhibit glycolysis. Hence, Met with JX06-NPs could excel the apoptosis of EC and impeding tumor growth [108].
Targeting hedgehog signaling pathway in prostate cancer cells by pbm nanoparticles
Prostate cancer (PCa) progression is caused by an alteration in the genome of the tumor growth [111]. Treating PCa is a significant problem as multiple challenges include drug resistance, failure of different treatments, and numerous molecular and clinical factors [112]. At the molecular level, the abnormal hedgehog (Hh) signaling pathway [113] play important role in prostate cancer development. It becomes the cause of a more aggressive and drug-resistant form of prostate cancer [114]. In normal and healthy cells, a variety of major cellular process including differentiation, development, growth and many others are regulating by Hh pathway. Furthermore, Hh play major role in the regeneration and tissue repair [115]. Human prostate cancer was found to have elevated expression of components in the Hh pathway, including glioma-associated oncogene homolog 1 (GLI-1) and sonic hedgehog (SHH) [116]. Thymoquinone (TQ), a natural chemical, was studied for its role in modulating Hh signalling in prostate cancer which was analyzed in the study [117]. In a study, planetary ball-milled nanoparticles (PBM-NPs) were generated using a natural polysaccharide [118]. It also contains TQ coated with an A10 RNA aptamer [118].
This aptamer's function is to bind to the membrane antigen identified as prostate-specific membrane antigen (PSMA) [119]. Docetaxel-resistant C4- 2B-R and LNCaP-R cells were prepared with higher expression of Hh and the integration of Hh signaling and drug resistance. A comparative study of the results, shown in (Figure 4) [120,121] found that A10-TQ-PBM-NPs were more effective in controlling the Hh pathway than free TQ. The study demonstrate that prostate cancer as well as Hh pathways are inhibited with the help of NPs based strategy [121].
Figure 4: For 48 hours, PC cells were treated with and without A10-conjugated TQ-NPs and assessment of ABCB1 (ATP Binding Cassette Subfamily B Member 1) protein expression by Western blots. Asterisks ** represent p values ≤ 0.01. UT: untreated (DTX-resistant); C: control (parent cells) [121].
The results showed that Hh signaling inhibition by TQ shows that cancers driven by Hh signaling can be treated with natural anticancer compounds. This natural approach is readily available, safe, and inexpensive. It was revealed that aptamer-based NPs with natural drugs efficiently reduced the drug concentration, bound to a particular target, and unloaded the drug to the specific cancer cells [122]. It also reveals that TQ regulate aberrant expression of the Hh pathway molecules in prostate cancer [123]. Moreover, the link between drug resistance and the Hh pathway and overexpression of ATP-binding cassette transporter genes reversed by TQ also became evident. A new strategy has been developed that utilizes aptamer-bound anticancer NPs for treating Hh signaling-driven cancers.
Application of Nanoparticles to Target mTOR Signaling Pathway
Serine/threonine kinase mammalian target of rapamycin (mTOR) controls and regulates cell growth and proliferation. under normal conditions [124]. In multiple cancers, mutated mTOR overexpression is found, and some targets of mTOR kinase signaling [125]. Hence, mTOR signaling has been identified as a potential target for anticancer therapy [126]. mTOR targeting inhibitors have more efficient and feasible pharmacological profiles than traditional anticancer drugs [127]. Moreover, these Inhibitors are generally easy to tolerate. It is important to mention that multiple NPs have shown optimostic ability to modify mTOR activity [[128]. The activity of mTOR metabolic pathways is summarized in the (Figure 5) [129]. As examples, amino-decorated NPs can consistently decrease mTOR activity while also promoting proliferation in leukaemia cell lines [130]. Blocking of mTOR signaling for treatment purpose has shown limited effectiveness [131]. For a varieties of tumors, NPs-based mTOR specific therapies put forward potential therapeutic options [132]. However, difficulties and progression are in parallel in the biomedical application of NPs [133]. The modification of mTOR activity is thoroughly investigated, the intricacy of cellular responses to functional NPs has been shown, and the fundamental obstacles in determining the molecular mechanism of the mTOR signaling pathway are discussed [134]. The study suggested potential therapeutic targets for cancer in the near future. The idea is proposed that sub cytotoxic doses of NPs could be used for inducing subcellular and metabolic alterations within the cell by the involvement of mTORC1 signaling [135]. Modulation of mTOR based on the therapeutic pupose of NPs can provide basic knowledge that can pave the way for the development of safe and effective NPs-based therapies targeting cancer metabolism [136].
Figure 5: Mammalian target of rapamycin (mTOR) signaling take place on the surface of lysosome. In favorable conditions for growth, the activities of Rag (Ras related GTPases) and Rheb (Ras homolog enriched in brain) GTPases cause the mTORC1 complex to be recruited and activated. When these inputs are disrupted, mTORC1 is inhibited. TSC is an abbreviation for tuberous sclerosis complex, while v-ATPase is an abbreviation for vacuolar- type H +-ATPase [129].
Suppression of ATP in cancer metabolism via pH activated mitochondria-targeted delivery of NPs
In a study, suppression of ATP in cancer metabolism by Nitric oxide NPs targeting pH-activated mitochondria-targeted delivery for metastasis prevention and drug resistance was observed. Mitochondria are commonly recognized as the powerhouse of the eukaryotic cells [137]. It has a vital role in the progression of tumors and other cancer-related important activities such as initiation, growth, and metastasis of cancer cells [138]. In cancer therapy, drug resistance is a major problem [139]. Overexpression of the protein P-glycoprotein, which is primarily responsible for this drug resistance and cassette of adenosine triphosphate (ATP) transporters on membranes of cancer cells which have the potential to continuously pump out the chemotherapeutics [140]. In this experiment, acid-activated mitochondria-targeted drug NPs were developed to smooth Nitric oxide delivery [141]. Nitric oxide has the starring role of overcoming ATP in cancer metabolism to enhance cancer treatment therapeutic effectiveness [142]. Combining acid-cleavable dimethyl maleic anhydride-modified PEG-linked mitochondria- targeting peptide and a-cyclodextrin (a-CD) was successful. These created NPs demonstrated better cellular absorption, extracellular tumor pH, and selective mitochondrial targeting, as well as an extended blood circulation duration. (6.5). Nitric oxide (NO) induces mitochondria dysfunction by enabling mitochondrial permeabilization of membrane and ATP downregulation. whereas the creation of tumor-derived macrovesicles for combating drug resistance and cancer spread might diminish P-glycoprotein-linked bioactivities. The genetic expression analysis represents that gene involved in the drug resistance and by precisely delivering nitric oxide to the mitochondria, metastasis can be tightly controlled [142]. It is a novel and pioneering research that acid-activated mitochondria-targeted nitric oxide NPs are suggested to be the antitumor and can provide potential insights for a wide range of NO-relevant cancer treatments [143].
Application of BPTES nanoparticles and metformin as combination therapy to target the cancer metabolic heterogeneity of cancer in the pancreas
There is currently no viable treatment for pancreatic cancer [144]. Treatment for pancreatic cancer is extremely difficult due to the presence of two different cancer cell populations [145]. For effective tumor control, it is necessary to target each group with a particular metabolic inhibitor. Glutamate is used by proliferating cells, and NPs containing inhibitors of glutamine metabolism can be efficiently induced to target proliferating cells [146]. Hypoxic cells, which divide slowly, are important in the metabolism of glucose [143]. Metformin, a medication used to treat diabetes, can be used to target them in an efficient manner. There is a need to investigate whether combination therapy can effectively enhance the survival of pancreatic cancer patients in clinical trials [147]. Combination therapy will be based on medicines that effectively limit the metabolism of glucose and glutamine [148]. Clinical experiments have used pharmacological suppression of the glutaminase enzyme to target one of the three major metabolisms of glutamine [149]. Although it is novel therapy, the current medications do not guarantee the greatest efficacy and safety. An emulsification technique has been employed in a study to encapsulate bis-2-(5- phenyl acetamido-1, 2, 4-thiadiazol-2-yl) ethyl sulfide (BPTES). BPTES is a selective and insoluble glutaminase enzyme inhibitor in nanoparticles. When compared to BPTES without encapsulation, BPTES NPs show improved pharmacokinetics and efficient clinical trials are currently using the glutaminase enzyme inhibitor CB-839. Unlike BPTES NPs, which have no such negative effect, it influences the plasma levels of liver enzymes. Pancreatic tumors from patients were transplanted into mouse models during the study. In these models, treatment with BPTES NPs significantly inhibits tumor growth. It was discovered that glutaminase inhibition, when applied in vivo with the HypoxCR reporter, inhibited tumor growth by concentrating on cancer cells that were actively reproducing. The hypoxic non-cycling cells were unaffected, though (Figure 6) from the metabolomics study illustrates how glycolysis and glycogen synthesis were necessary for the survival of cells after glutaminase enzyme inhibition [149]. These results led to the use of metformin and BPTES nanoparticles for combination therapy. Compared to the treatment alone, it caused a very large reduction in pancreatic cancer cases. Thus, a unique approach for treating pancreatic cancer involves targeting several cancer metabolic pathways, including glucose metabolism using nanoparticle-based medication delivery [149].
Figure 6: Tumors from mice given BPTES-NPs were subjected to metabolomics analysis. (A-C, Top) The orange titles represent the 1 H13C NMR spectra of lactate generated from labeled glucose or glutamine as well as 13C- labeled glutamine, glucose, and lactate. Each 1 H peak originated from protons that were firmly bound to 13C, and the peak designation designates the 13C. (4) (A-C, Bottom) Relative peak intensities of [13C] glutamine (A), [13C] glucose (and isomers) (B), and [13C] lactate (C) overall isotopomers normalized by tumor wet weight. (D) Ratio of lactate to glucose in tumors of mice given either blank-NPs (blue bars) or BPTES-NPs (red bars) [146].
Challenges and future perspectives in clinical applications of nanoparticles-based cancer treatments
Knowledge and research in nanotechnology have hiked in recent years. But the rate of translating nanotechnology into clinical trials is still limited [149]. Only a few clinical trials could become successful whereas most of them could not cross the in vivo and in vitro stages [150]. Each NP- based cancer treatment has distinct challenges in its clinical translation, but most nanoparticle formulations face similar challenges [151]. These challenges can be either biological, technological, or study-design related. In biological challenges, the major challenges include an absence of routes of administration NPs, their unstable biodistribution, degradation, and toxicity [152]. Nanoparticles are intravenously injected directly into blood which makes it challenging to stay and interact with the target sites. Magnetic NPs can be used as an alternative for the controlled delivery of the NPs but the effects of magnetic fields on the human body are still to be explored and researched [153]. Introducing NPs into the human body requires a lot of focus and care. Although NPs are made as per standards of safety and modified to increase the half-life and retention time, there is the risk of lung, kidney, and liver damage [154]. Some studies have shown high deposition of NPs in the lungs along with inflammatory and cytotoxic effects. Factors that affect toxicity include particle size, shape, surface area, solubility, and agglomeration [155]. Research has revealed that NPs could also be the cause of the generation of free radicals that ultimately damage healthy cells [156]. To overcome this challenge, there is a need for more biocompatible substances, such as chitosan, that can disintegrate after infrared light irradiation [157]. The mononuclear phagocytic system (MPS) is yet another significant obstacle to the use of NPs in the treatment of cancer [158]. NPs adsorb proteins to produce plasma cells. These plasma cells target MPS for the uptake of NPS [159]. In some experiments, NPs were coated with materials that inhibit the synthesis of the protein to avoid escaping the MPS system, but the results were not significant [160]. With adequate research and experimental investigations, designing NPs that target macrophages can be utilized as a substitute [161]. Currently, tactics include blocking CD47-SIRP pathways, preventing macrophage recruitment, reprogramming and eliminating tumor-associated macrophages (TAMs), and reducing TAMs [162]. Scaled-up synthesis of NPs, optimization and performance prediction of NPs are technological challenges that are acting as hurdles in the translation of NPs into clinical trials [163]. The NPs being used in in vivo and in vitro studies are majorly produced in minor batches as high quantity production is not constantly feasible due to certain factors [164]. Hence, the best animals are not systematically designed and optimized. To curtail this challenge, nanoformulations can be tested by selective iterations for a single optimized formulation [165]. It should be first tested in animal models before introducing to human beings. Furthermore, the prediction of NP's efficacy and performance in human trials is a tough task. For this, computational and theoretical modeling along with experimental results can be designed to emulate physiological tissues and surroundings [166]. For instance, organs-on-chips technology can be utilized for the improvement in the prediction and efficacy of NPs performance [167]. Moreover, The inability of successful NP-based clinical studies is significantly attributed to problems with study design [168]. Size and timing of NPs therapy are two difficulties in study design. The majority of the experimental studies of NPs use animal and cell models. In human trials, it might not produce believable results [169]. Additionally, as metastasis is a crucial characteristic of tumors, there is a need for adequate study on cancer metastasis models [170]. When developing NP-based cancer therapeutics, genetic, prior medical history, and environmental factors must be taken into account [171]. Drug resistance is still another significant obstacle to NPs-based cancer therapy. Many nanoformulations are approved for use but never used as first-line therapies but are saved for further treatments on the stages of cancer where progression is found in the clinical trials scenario. The patients have been either treated with multiple lines of therapy or have developed drug resistance [172]. These challenges become the reason for fewer chances of success of NPs-based treatments for cancer.
Conclusions
For cancer experts, cancers have become a significant concern because they claim a lot of lives and show resistance to cancer treatment methods. Cancer is very challenging to treat because, for example, cancer cells are multidrug-resistant, relapse, and metastatic. In several branches of science and technology, nanotechnology, which is still in its infancy, has proven to assist. The potential of NPs for cancer therapy has also been studied by researchers who specialize in cancer. Researchers have looked at and studied several cancer cures using nanoparticles. All nano-based treatments target cancer metabolic pathways to curtail the uncontrolled growth of tumor cells. Numerous strategies have been applied, studied, and analyzed, such as encapsulating drugs with NPs, targeting glucose metabolism in tumor cells, enzyme-responsible for cancer metabolism, and many others. All these cancer-treating strategies came out with promising results and opened the gates of potential future perspectives regarding cancer therapies. Studies showed that NPs-based cancer therapies ensure more efficiency in drug delivery, efficacy, and stability. Furthermore, NP-based treatments are easily accessible, inexpensive, and have few long-term negative effects. The FDA has approved several Nanomedicines that are successfully giving cancer patients new leases of life. NPs-based cancer therapeutics still need a lot of development to be effective in clinical settings. Animal models like mice are used for the majority of investigations, with good results. The encouraging findings encourage researchers studying cancer to continue investigating various NP types, their therapeutic potential for focusing on cancer metabolism, problems they may face, and solutions to those challenges.
Ethics Approval and Consent to Participate
This does not involve any animals or humans’ studies.
Consent for Publication
All authors approved the final version of manuscript.
Availability of Data and Materials
Not applicable.
Competing Interest
All the authors declare that they have no financial or any other competing interests.
Authors’ Contribution
Zia Ud Din and Yasmeen Khan prepared the draft and wrote the manuscript. Nasira Anbreen helped in preparing the figures. Amn Zia refined and arranged the contents of the manuscript. All authors approved the final manuscript.
References
- https://apps.who.int/iris/bitstream/handle/10665/41864/0965546608_eng.pdf
- Yadav AR, Mohite SK. (2020) Cancer-A silent killer: An overview. Asian J. Pharm. Res. 10(3):213–16.
- Epstein T, Gatenby RA, Brown JS. (2017) The Warburg effect as an adaptation of cancer cells to rapid fluctuations in energy demand. PLoS One. 12(9):e0185085.
- Benjamin DI, Cravatt BF, Nomura DK. (2012) Global profiling strategies for mapping dysregulated metabolic pathways in cancer. Cell Metab.16(5):565–77.
- Rho O, Kim DJ, Kiguchi K, DiGiovanni J. (2011) Growth factor signaling pathways as targets for prevention of epithelial carcinogenesis. Mo Carcinog. 50(4):264–79.
- K. Dzobo, D. A. Senthebane, C. Ganz, N. E. Thomford, A. Wonkam, and C. Dandara, “Advances in therapeutic targeting of cancer stem cells within the tumor microenvironment: an updated review. Cells. 9(8):1896.
- Christofk HR, Vander Heiden MG, Harris MH, Ramanathan A, Gerszten RE, et al. (2008) The M2 splice isoform of pyruvate kinase is important for cancer metabolism and tumor growth. Nature. 452(7184):230-33.
- Marin JJG, Al-Abdulla R, Lozano E, Briz O, Bujanda L. (2016) Mechanisms of resistance to chemotherapy in gastric cancer. Anticancer Agents Med Chem. 16(3):318–34.
- Goldie JH, Coldman AJ. (1984) The genetic origin of drug resistance in neoplasms: implications for systemic therapy. Cancer Res. 44(9):3643–53.
- M. Prato, K. Kostarelos, and A. Bianco. (2008) Functionalized carbon nanotubes in drug design and discovery. Acc Chem Res. 41(1):60–68.
- Páez D, Labonte MJ, Bohanes P, Zhang W, Benhanim L. (2012) Cancer dormancy: a model of early dissemination and late cancer recurrence. Clin Cancer Res. 18(3):645–53.
- Kirschner D, Panetta JC. (1998) Modeling immunotherapy of the tumor–immune interaction. J Math Biol. 37(3):235–52.
- Suppes T, Baldessarini RJ, Faedda GL, Tohen M. (1991) Risk of recurrence following discontinuation of lithium treatment in bipolar disorder. Arch Gen Psychiatry. 48(12):1082–88.
- Sadeghi M, Sadeghi S, Naghib SM, Garshasbi HR. (2023) A Comprehensive Review on Electrochemical Nano Biosensors for Precise Detection of Blood-Based Oncomarkers in Breast Cancer. Biosensors. 13(4):481.
- Schmidt CK, Medina-Sánchez M, Edmondson RJ, Schmidt OG. (2020) Engineering microrobots for targeted cancer therapies from a medical perspective. Nat Commun. 11(1):5618.
- Olusanya TOB, Haj Ahmad RR, Ibegbu DM, Smith JR, Elkordy AA. (2018) Liposomal drug delivery systems and anticancer drugs. Molecules. 23(4):907.
- Yan Y, Kumar AB, Finnes H, Markovic SN, Park S, et al. (2018) Combining immune checkpoint inhibitors with conventional cancer therapy. Front. Immunol. 9:1739.
- Sullivan M, Bouffet E, Rodriguez-Galindo C, Luna-Fineman S, Khan MS, et al. (2020) The COVID‐19 pandemic: a rapid global response for children with cancer from SIOP, COG, SIOP‐E, SIOP‐PODC, IPSO, PROS, CCI, and St Jude Global. Pediatr Blood Cancer. 67(7):e28409.
- Yavarpour-Bali H, Ghasemi-Kasman M, Pirzadeh M. (2019) Curcumin-loaded nanoparticles: A novel therapeutic strategy in treatment of central nervous system disorders. Int. J. Nanomedicine. 14:4449–60.
- Valenzuela C, Chen C, Sun M, Ye Z, Zhang J. (2021) Strategies and applications of covalent organic frameworks as promising nanoplatforms in cancer therapy. J Mater Chem B. 9(16):3450– 83.
- Aydın A, Sipahi H, Charehsaz M. (2012) Nanoparticles toxicity and their routes of exposures. Rec Adv Nov drug Carr Syst. 483–500.
- Ion RM, Sorescu AA, Nuta A. (2021) Green synthesis of lanthanides and actinides-based nanomaterials,” in Handbook of Greener Synthesis of Nanomaterials and Compounds. Elsevier. 2:355–88.
- Liu Y, Yin J, Nie Z. (2015) Self‐Assembly of Amphiphilic Block Copolymer‐Tethered Nanoparticles: a New Approach to Nanoscale Design of Functional Materials. Macromol Rapid Commun. 36(8):711–25.
- Asha AB, Narain R. (2020) Nanomaterials properties in Polymer science and nanotechnology. Elsevier. 343–59.
- Dirgantari PD, Nandiyanto ABD, Machmud A. (2019) Development strategy of earthworms nanoparticle products using ball mill methods in Indonesia. J Eng Sci Technol. 14(2):589– 98.
- Riley RS, Day ES. (2017) Gold nanoparticle‐mediated photothermal therapy: applications and opportunities for multimodal cancer treatment. Wiley Interdiscip Rev Nanomed Nanobiotechnol. 9(4):10.
- Sztandera K, Gorzkiewicz M, Klajnert-Maculewicz B. (2018) Gold nanoparticles in cancer treatment. Mol Pharm. 16(1):1–23.
- Zhang JIN, Lan CQ, Post M, Simard B, Deslandes Y, et al. (2006) Design of nanoparticles as drug carriers for cancer therapy. Cancer Genomics Proteomics. 3(3–4):147–57.
- Hou YJ, Yang XX, Liu RQ, Zhao D, Guo CX, et al. (2020) Pathological mechanism of photodynamic therapy and photothermal therapy based on nanoparticles. Int J Nanomedicine. 15:6827–38.
- Anquez F, Yazidi‐Belkoura IE, Randoux S, Suret P, Courtade E. (2012) Cancerous cell death from sensitizer free photoactivation of singlet oxygen. Photochem. Photobiol. 88(1):167-74.
- Yang M, Deng J, Su H, Gu S, Zhang J, et al. (2021) Small organic molecule-based nanoparticles with red/near-infrared aggregation-induced emission for bioimaging and PDT/PTT synergistic therapy,” Mater. Chem. Front. 5(1):406–17.
- Shao D, Wang Z, Chang Z, Chen L, W.-F. Dong, and K. W. Leong, “Janus metallic mesoporous silica nanoparticles: Unique structures for cancer theranostics. Curr Opin Biomed Eng. 19:100294.
- Arruebo M, Fernández-Pacheco R, Ibarra MR, Santamaría J. (2007) Magnetic nanoparticles for drug delivery. Nano Today. 2(3):22-32.
- Zaimy MA, Saffarzadeh N, Mohammadi A, Pourghadamyari H, Izadi P, et al. (2017) New methods in the diagnosis of cancer and gene therapy of cancer based on nanoparticles. Cancer Gene Ther. 24(6):233–43.
- Attia MF, Anton N, Wallyn J, Omran Z, Vandamme TF. (2019) An overview of active and passive targeting strategies to improve the nanocarriers efficiency to tumour sites. J Pharm Pharmacol. 71(8):1185-98.
- Mercier MC, Dontenwill M, Choulier L. (2017) Selection of nucleic acid aptamers targeting tumor cell- surface protein biomarkers. Cancers. 9(6):69.
- Misra R, Sahoo SK. (2010) Intracellular trafficking of nuclear localization signal conjugated nanoparticles for cancer therapy. Eur J Pharm Sci. 39(1–3):152–63.
- Pishavar E, Luo H, Bolander J, Atala A, Ramakrishna S. (2021) Nanocarriers, progenitor cells, combinational approaches, and new insights on the retinal therapy. Int J Mol Sci. 22(4):1776.
- Vander Heiden MG. (2011) Targeting cancer metabolism: a therapeutic window opens. Nat Rev Drug Discov. 10(9):671–84.
- Chavanpatil MD, Khdair A, Panyam J. (2006) Nanoparticles for cellular drug delivery: mechanisms and factors influencing delivery. J Nanosci Nanotechnol. 6( 9–10):2651–63.
- Kedar U, Phutane P, Shidhaye S, Kadam V. (2010) Advances in polymeric micelles for drug delivery and tumor targeting. Nanomedicine. 6(6):714–29.
- Yoo J, Park C, Yi G, Lee D, Koo H, et al. (2019) Active targeting strategies using biological ligands for nanoparticle drug delivery systems. Cancers. 11(5):640.
- Pérez-Herrero E, Fernández-Medarde A. (2015) Advanced targeted therapies in cancer: Drug nanocarriers, the future of chemotherapy. Eur J Pharm Biopharm. 93952):79.
- Bahrami B, Hojjat-Farsangi M, Mohammadi H, Anvari E, Ghalamfarsa G, et al. (2017) Nanoparticles and targeted drug delivery in cancer therapy. Immunol Lett. 190: 64–83.
- Wei QY, Xu YM, Lau ATY. (2020) Recent progress of nanocarrier-based therapy for solid malignancies. Cancers (Basel). 12(10):2783.
- Anjum S, Hashim M, Malik SS, Khan M, Lorenzo JM, et al. (2021) Recent advances in zinc oxide nanoparticles (ZnO NPs) for cancer diagnosis, target drug delivery, and treatment. Cancers (Basel). 13(18):4570.
- Kong N, Zhang R, Wu G, Sui X, Wang J, et al. (2022) Intravesical delivery of KDM6A-mRNA via mucoadhesive nanoparticles inhibits the metastasis of bladder cancer. Proc Natl Acad Sci U S A. 119(7):e2112696119.
- Cheng Z, Li M, Dey R, Chen Y. (2021) Nanomaterials for cancer therapy: Current progress and perspectives. J Hematol Oncol.14(1):1–27.
- Jones RG, Thompson CB. (2009) Tumor suppressors and cell metabolism: a recipe for cancer growth. Genes Dev. 23(5):537–48.
- Singh P, Kumari S, Guldhe A, Misra R, Rawat I, et al. (2016) Trends and novel strategies for enhancing lipid accumulation and quality in microalgae. Renew Sustain Energy Rev. 55:1–16.
- Sanz EA, Siles MG, Fernández LR, Roldán RV, Domínguez AR, et al. (2019) Nutritional risk and malnutrition rates at diagnosis of cancer in patients treated in outpatient settings: Early intervention protocol. Nutrition. 57:148–53.
- Kreuzaler P, Panina Y, Segal J, Yuneva M. (2020) Adapt and conquer: Metabolic flexibility in cancer growth, invasion and evasion. Mol Metab. 33:83–101.
- Nagao A, Kobayashi M, Koyasu S, Chow CCT, Harada H. (2019) HIF-1-dependent reprogramming of glucose metabolic pathway of cancer cells and its therapeutic significance. Int J Mol Sci. 20(2):238.
- Ward PS, Thompson CB. (2012) Signaling in control of cell growth and metabolism. Cold Spring Harb Perspect Biol. 4(7):a006783.
- Spinelli JB, Haigis MC. (2018) The multifaceted contributions of mitochondria to cellular metabolism. Nat Cell Biol. 20:(7):745–54.
- Zhu J, Thompson CB. (2019) Metabolic regulation of cell growth and proliferation. Nat Rev Mol cell Biol. 20(7):436–50.
- Hao Y, Samuels Y, Li Q, Krokowski D, Guan BJ, et al. (2016) Oncogenic PIK3CA mutations reprogram glutamine metabolism in colorectal cancer. Nat Commun. 7:11971.
- Michalovitz D, Halevy O, Oren M. (1990) Conditional inhibition of transformation and of cell proliferation by a temperature-sensitive mutant of p53. Cell. 62(4):671–80.
- Wieman HL, Wofford JA, Rathmell JC. (2007) Cytokine stimulation promotes glucose uptake via phosphatidylinositol-3 kinase/Akt regulation of Glut1 activity and trafficking. Mol Biol Cell. 18(4):1437–46.
- Gaglio D, Metallo CM, Gameiro PA, Hiller K, Danna LS, et al. (2011) Oncogenic K‐Ras decouples glucose and glutamine metabolism to support cancer cell growth. Mol Syst Biol. 7(1):523.
- Hofmann JW, Zhao X, Cecco MD, Peterson AL, Pagliaroli L, et al. (2015) Reduced expression of MYC increases longevity and enhances healthspan. Cell. 160(3):477–88.
- Zheng JIE. (2012) Energy metabolism of cancer: Glycolysis versus oxidative phosphorylation. Oncol Lett. 4(6):1151–57.
- Miller DM, Thomas SD, Islam A, Muench D, Sedoris K. (2012) c-Myc and cancer metabolism. Clin cancer Res. 18(20):5546–53.
- Dang CV. (2010) Rethinking the Warburg effect with Myc micromanaging glutamine metabolism. Cancer Res. 70(3):859–62.
- Filomeni G, De Zio D, Cecconi F. (2015) Oxidative stress and autophagy: the clash between damage and metabolic needs. Cell Death Differ. 22(3):377–88.
- Jiang J, Srivastava S, Zhang J. (2019) Starve cancer cells of glutamine: break the spell or make a hungry monster. Cancers. 11(6):804.
- Yizhak K, Chaneton B, Gottlieb E, Ruppin E. (2015) Modeling cancer metabolism on a genome scale. Mol Syst Biol. 11(6):817.
- Kamphorst JJ, Nofal M, Commisso C, Hackett SR, Lu W, et al. (2015) Human pancreatic cancer tumors are nutrient poor and tumor cells actively scavenge extracellular protein. Cancer Res. 75(3):544–53.
- Finicle BT, Jayashankar V, Edinger AL. (2018) Nutrient scavenging in cancer. Nat Rev Cancer. 18(10):619-33.
- Efeyan A, Zoncu R, Sabatini DM. (2012) Amino acids and mTORC1: from lysosomes to disease,” Trends Mol. Med. 18(9):524–33.
- Xiao F, Li J, Huang K, Li X, Xiong Y, et al. (2021) Macropinocytosis: mechanism and targeted therapy in cancers. Am J Cancer Res. 11(1):14-30.
- Kieliszek M, Błażejak S, Bzducha-Wróbel A, Kot AM. (2019) Effect of selenium on lipid and amino acid metabolism in yeast cells. Biol Trace Elem Res. 187(1):316–27.
- Olivares O, Mayers JR, Gouirand V, Torrence ME, Gicquel T, et al. (2017) Collagen-derived proline promotes pancreatic ductal adenocarcinoma cell survival under nutrient limited conditions. Nat Commun. 8(1):16031.
- Yang L, Moss T, Mangala LS, Marini J, Zhao H, et al. (2014) Metabolic shifts toward glutamine regulate tumor growth, invasion and bioenergetics in ovarian cancer. Mol Syst Biol. 10(5):728.
- Davidson SM, Jonas O, Keibler MA, Hou HW, Luengo A, et al. (2017) Direct evidence for cancer-cell-autonomous extracellular protein catabolism in pancreatic tumors. Nat Med. 23(2):235–41.
- Recouvreux MV, Commisso C. (2017) Macropinocytosis: a metabolic adaptation to nutrient stress in cancer. Front Endocrinol(Lausanne). 8:261.
- Lambies G, Commisso C. (2022) Macropinocytosis and cancer: From tumor stress to signaling pathways. Subcell Biochem. 98:15–40.
- Munir R, Lisec J, Swinnen JV, Zaidi N, (2019) Lipid metabolism in cancer cells under metabolic stress. Br J Cancer. 120(12):1090–98.
- Menendez JA, Mehmi I, Atlas E, Colomer R, Lupu R. (2004) Novel signaling molecules implicated in tumor-associated fatty acid synthase-dependent breast cancer cell proliferation and survival: Role of exogenous dietary fatty acids, p53-p21WAF1/CIP1, ERK1/2 MAPK, p27KIP1, BRCA1, and NF-κB. Int J Oncol. 24(3):591–8.
- LeBoeuf SE, Wu WL, Karakousi TR, Karadal B, Jackson SR, et al. (2020) Activation of oxidative stress response in cancer generates a druggable dependency on exogenous non-essential amino acids. Cell Metab. 31(2):339–50.
- White E. (2013) Exploiting the bad eating habits of Ras-driven cancers. Genes Dev. 27(19):2065.
- Fuentes NR. (2017) Plasma Membrane Lipid Therapy: Disruption of Oncogenic Ras Driven Phenotypes by Membrane Targeted Dietary Bioactives (Mtdb). 77(13):179.
- Kim SM, Nguyen TT, Ravi R, Kubiniok P, Finicle BT, et al. (2018) PTEN deficiency and AMPK activation promote nutrient scavenging and anabolism in prostate cancer cells. Cancer Discov. 8(7):866–83.
- Strong AL, Ohlstein JF, Biagas BA, Rhodes LV, Pei DT, et al. (2015) Leptin produced by obese adipose stromal/stem cells enhances proliferation and metastasis of estrogen receptor positive breast cancers. Breast Cancer Res. 17(1):112.
- Nag S, Patel S, Mani S, Hussain T. (2013) Role of Angiotensin II Type 2 Receptor in Regulation of Lipid Metabolism and Adiposity. Mol Cell Biochem. 46(1-2):195-204.
- Schug ZT, Vande Voorde J, Gottlieb E. (2016) The metabolic fate of acetate in cancer. Nat Rev Cancer. 16(11):708–17.
- Wick W, Pope W, Hegi M, Soffietti R, Aldape K. (2015) Highlights from the Literature. Neuro Oncol. 18(1):9–11.
- Bakalova R, Zhelev Z, Aoki I, Saga T. (2013) Tissue redox activity as a hallmark of carcinogenesis: from early to terminal stages of cancer. Clin Cancer Res. 19(9):2503–17.
- Mashimo T, Pichumani K, Vemireddy V, Hatanpaa KJ, Singh DK, et al. (2014) Acetate is a bioenergetic substrate for human glioblastoma and brain metastases. Cell. 159(7):1603–14.
- Corbet C, Feron O. (2015) Metabolic and mind shifts: from glucose to glutamine and acetate addictions in cancer. Curr Opin Clin Nutr Metab Care. 18(4):346–53.
- Salzillo T, Hu J, Nguyen L, Whiting N, Lee J, et al. (2016) Interrogating metabolism in brain cancer,” Magn Reson Imaging Clin. 24(4):687–3.
- Mandal PK, Roy RG, Samkaria A, Maroon JC, Arora Y. (2022) In vivo 13C magnetic resonance spectroscopy for assessing brain biochemistry in health and disease. Neurochem Res. 47(5):1183–01.
- Huang Z, Zhang M, Plec AA, Esatill SJ, cai L, et al. (2018) ACSS2 promotes systemic fat storage and utilization through selective regulation of genes involved in lipid metabolism. Proc Natl Acad Sci. 115(40):E9499–E9506.
- Comerford SA, Huang Z, Du X, Wang Y, Cai L, et al. (2014) Acetate dependence of tumors. Cell. 159(7):1591–02.
- Zhao S, Torres AM, Henry RA, Trefely S, Trefely S, et al. (2016) ATP-citrate lyase controls a glucose-to-acetate metabolic switch. Cell Rep.17(4):1037–52.
- Bidkhori G, Benfeitas R, Klevstig M, Zhang C, Nielsen J, et al. (2018) Metabolic network-based stratification of hepatocellular carcinoma reveals three distinct tumor subtypes. Proc Natl Acad Sci U S A. 115(50):E11874:883.
- Ligorio F, Pellegrini I, Castagnoli L, Vingiani A, Lobefaro R, et al. (2021) Targeting lipid metabolism is an emerging strategy to enhance the efficacy of anti-HER2 therapies in HER2-positive breast cancer,” Cancer Lett. 511:77–87.
- Olson Ka, Schell JC, Rutter J. (2016) Pyruvate and metabolic flexibility: illuminating a path toward selective cancer therapies. Trends Biochem Sci. 41(3):219–30.
- Clark PM, Ebiana VA, Gosa L, Cloughesy TF, Nathanson DA. (2017) Harnessing preclinical molecular imaging to inform advances in personalized cancer medicine. J Nucl Med. 58(5): 689-96.
100. Gentry-Maharaj A, Karpinskyj C. (2020) Current and future approaches to screening for endometrial cancer. Best Pract Res Clin Obstet Gynaecol. 65:79-97.
101. https://eprints.qut.edu.au/200151/
102. Fischer MA, Stedman MR, Lii J, Vogeli C, Shrank WH, et al. (2010) Primary medication non-adherence: analysis of 195,930 electronic prescriptions. J Gen Intern Med. 25(4):284–90.
103. Radhouane K, Bedioui A, Yedeas MD, Zayet S, Jebari M, et al. (2020) Brain abscess due to Candida glabrata in an immunocompetent patient. A case report with update and literature review. IDCases. 22:e00996.
104. Byrne FL, Martin AR, Kosasih M, Caruana BT, Farrell R. (2020) The role of hyperglycemia in endometrial cancer pathogenesis. Cancers (Basel). 12(5):1191.
105. Meng F, Hennink WE, Zhong Z. (2009) Reduction-sensitive polymers and bioconjugates for biomedical applications. Biomaterials. 30(12):2180–98.
106. Amin S, Lux A, O’Callaghan F. (2019) The journey of metformin from glycaemic control to mTOR inhibition and the suppression of tumour growth. Br J Clin Pharmacol. 85(1):37–46.
107. Pusch W, Flocco MT, Leung SM, Thiele H, Kostrzewa M. (2003) Mass spectrometry-based clinical proteomics. Pharmacogenomics. 4(4):463–76.
108. Kawano Y, Sasano T, Arima Y, Kushima S, Tsujita K, et al. (2022) A novel PDK1 inhibitor, JX06, inhibits glycolysis and induces apoptosis in multiple myeloma cells. Biochem Biophys Res Commun. 587:153–59.
109. Ren S, Wei GH, Liu D, Wang L, Hou Y, et al. (2018) Whole-genome and transcriptome sequencing of prostate cancer identify new genetic alterations driving disease progression. Eur Urol.73(3):322–39.
110. Yang X, Cheng Y, Zhou J, Zhang L, Li X, et al. (2022) Targeting cancer metabolism plasticity with JX06 nanoparticles via inhibiting PDK1 combined with met form in for endometrial cancer patients with diabetes. Adv Sci. 9(8):2104472.
111. Semenas J, Allegrucci C, Boorjian SA, Mongan NP, Persson JL. (2012) Overcoming drug resistance and treating advanced prostate cancer. Curr Drug Targets. 13(10):1308–23.
112. Patel H, Joshi J, Desai U, Raval A, Shah F. (2018) Hedgehog Signaling: An Emerging Targeting Therapy in Cancer. Asian J Pharm Pharmacol. 4(5):535–45.
113. Hassounah NB, Bunch TA, McDermott KM. (2012) Molecular pathways: the role of primary cilia in cancer progression and therapeutics with a focus on Hedgehog signaling. Clin Cancer Res. 18(9):2429–35.
114. Fuchs Y, Steller H, (2011) Programmed cell death in animal development and disease. Cell. 147(4):742–58.
115. Singh SK, Gordetsky JB, Bae S, Acosta EP, Lillard Jr JW, et al. (2020) Selective targeting of the hedgehog signaling pathway by PBM nanoparticles in docetaxel-resistant prostate cancer. Cells. 9(9):1976.
116. Niyaz M, Khan MS, Mudassar S. (2019) Hedgehog signaling: an Achilles’ heel in cancer. Transl Oncol. 12(10):1334–44.
117. El-Far AH, Tantawy MA, Al Jaouni SK, Mousa SA. (2020) Thymoquinone-chemotherapeutic combinations: new regimen to combat cancer and cancer stem cells. Naunyn Schmiedebergs Arch Pharmacol. 393(9):1581–98.
118. Wang X, Zhou Q, Li X, Gan X, Liu P, et al. (2022) Insights into Aptamer–Drug Delivery Systems against Prostate Cancer. Molecules. 27(11):3446.
119. Rockey WM, Hernandez FJ, Huang SY, Cao S, Howell CA, et al. (2011) Rational truncation of an RNA aptamer to prostate-specific membrane antigen using computational structural modeling. Nucleic Acid Ther. 21(5):299–14.
120. Lima TS, Gato DI, Souza LD, Stenvang J, Lima DS, et al. (2021) Molecular profiling of docetaxel-resistant prostate cancer cells identifies multiple mechanisms of therapeutic resistance. Cancers (Basel). 13(6):1290.
121. Mourtada-Maarabouni M, Hasan MA, Farzaneh F, Williams GT. (2010) Inhibition of human T-cell proliferation by mammalian target of rapamycin (mTOR) antagonists requires noncoding RNA growth- arrest-specific transcript 5 (GAS5). Mol Pharmacol. 78(1):19–28.
122. Sathiyaseelan A, Saravanakumar K, Mariadoss AVA, Wang MH. (2021) pH-controlled nucleolin targeted release of dual drug from chitosan-gold based aptamer functionalized nano drug delivery system for improved glioblastoma treatment. Carbohydr Polym. 262:117907.
123. Singh R. (2019) M. S. of M. I. A. U. States, “Repurposing Thymoquinone as Therapy for Metastatic Castration-Resistant Prostate Cancer. Morehouse Sch Med Inc. Atlanta United States.
124. Polivka Jr J, Janku F. (2014) Molecular targets for cancer therapy in the PI3K/AKT/mTOR pathway.
Pharmacol Ther. 142(2):164–75.
125. Meric-Bernstam F, Gonzalez-Angulo AM. (2009) Targeting the mTOR signaling network for cancer therapy. J Clin Oncol. 27(13):2278.
126. Dancey JE, Chen HX. (2006) Strategies for optimizing combinations of molecularly targeted anticancer agents. Nat Rev Drug Discov. 5(8):649–59.
127. Cordani M, Somoza A. (2019) Targeting autophagy using metallic nanoparticles: A promising strategy for cancer treatment. Cell Mol life Sci. 76:1215–42.
128. Lunova M, Smolkova B, Lynnyk A, Uzhytchak M, Jirsa M, et al. (2019) Targeting the mTOR signaling pathway utilizing nanoparticles: A critical overview Cancers (Basel). 11(1):82.
129. Watanabe R, Wei L, Huang J. (2011) mTOR signaling, function, novel inhibitors, and therapeutic targets. J Nucl Med. 52(4):497–500.
130. Mendes R, Carreira B, Baptista PV, Fernandes AR. (2016) Non-small cell lung cancer biomarkers and targeted therapy-two faces of the same coin fostered by nanotechnology. Expert Rev Precis Med drug Dev. 1(2):155-68.
131. Cardoso VF, Francesko A, Ribeiro C, Bañobre‐López M, Martins P, et al. (2018) Advances in magnetic nanoparticles for biomedical applications. Adv Healthc Mater. 7(5):1700845.
132. Halpern BS, Frazier M, Afflerbach J, Lowndes JS, Micheli F, et al. (2019) Recent pace of change in human impact on the world’s ocean. Sci Rep. 9(1):11609.
133. Deng Y, Jia F, Chen X, Jin Q, Ji J. (2020) ATP suppression by pH‐activated mitochondria‐targeted
delivery of nitric oxide nanoplatform for drug resistance reversal and metastasis inhibition. Small. 16(23):2001747.
134. Conroy T, Bachet JB, Ayav A, Huguet F, Lambert A, et al. (2016) Current standards and new innovative approaches for treatment of pancreatic cancer. Eur J Cancer. 57:10–22.
135. Liu N, Tang M. (2020) Toxic effects and involved molecular pathways of nanoparticles on cells and subcellular organelles. J Appl Toxicol. 40(1):16-36.
136. Hermann PC, Huber SL, Herrler T, Aicher A, Elwart JW, et al. (2007) Distinct populations of cancer stem cells determine tumor growth and metastatic activity in human pancreatic cancer. Cell Stem Cell. 1(3):313–23.
137. Javadov S, Kuznetsov AV. (2013) Mitochondria: the cell powerhouse and nexus of stress. Front Physiol. 4:207.
138. Yin F, Cadenas E. (2015) Mitochondria: the cellular hub of the dynamic coordinated network. Antioxid Redox Signal. 22(12):961-64.
139. Nikolaou M, Pavlopoulou A, Georgakilas AG, Kyrodimos E. (2018) The challenge of drug resistance in cancer treatment: a current overview. Clin Exp Metastasis. 35:309–18.
140. Borst P, Evers R, Kool M, Wijnholds J. (2000) A family of drug transporters: the multidrug resistance- associated proteins. J Natl Cancer Inst. 92(16):1295–02.
141. Sun W, Luan S, Qi C, Tong Q, Yan S, et al. (2019) Aspulvinone O, a natural inhibitor of GOT1 suppresses pancreatic ductal adenocarcinoma cells growth by interfering glutamine metabolism. Cell Commun Signal. 17:1–11.
142. Martinez-Outschoorn UE, Peiris-Pagés M, Pestell RG, Sotgia F, Lisanti MP. (2017) Cancer metabolism: a therapeutic perspective. Nat Rev Clin Oncol. 14(1):11–31.
143. Kathagen-Buhmann A, Schulte A, Weller J, Holz M, Herold-Mende C, et al. (2016) Glycolysis and the pentose phosphate pathway are differentially associated with the dichotomous regulation of glioblastoma cell migration versus proliferation. Neuro Oncol. 18(9):1219–29.
144. Dimcevski G, Kotopoulis S, Bjanes T, Hoem D, Schjott J, et al. (2016) A human clinical trial using ultrasound and microbubbles to enhance gemcitabine treatment of inoperable pancreatic cancer. J Control Release. 243:172–81.
145. Tanaka K, Sasayama T, Irino Y, Takata K, Nagashima H, et al. (2015) Compensatory glutamine metabolism promotes glioblastoma resistance to mTOR inhibitor treatment. J Clin Invest. 125(4):1591–02.
146. Elgogary A, Xu Q, Poore B, Alt J, Zimmermann SC, et al. (2016) Combination therapy with BPTES nanoparticles and metformin targets the metabolic heterogeneity of pancreatic cancer. Proc Natl Acad Sci. 113(36):E5328–336.
147. Kourelis TV, Siegel RD. (2012) Metformin and cancer: new applications for an old drug. Med Oncol. 29:1314–27.
148. Xu Y, Yu Z, Fu H, Guo Y, Hu P, et al. (2022) Dual inhibitions on glucose/glutamine metabolisms for nontoxic pancreatic cancer therapy. ACS Appl Mater Interfaces. 14(19):21836–847.
149.nUdupa S, Nguyen S, Hoang G, Nguyen T, Quinones A, et al. (2019) Upregulation of the glutaminase II pathway contributes to glutamate production upon glutaminase 1 inhibition in pancreatic cancer. Proteomics. 19(21–22):1800451.
150. Archetti M, Ferraro DA, Christofori G. (2015) Heterogeneity for IGF-II production maintained by public goods dynamics in neuroendocrine pancreatic cancer. Proc Natl Acad Sci. 112(6):1833–38.
151.vBae YH, Park K. (2020) Advanced drug delivery 2020 and beyond: Perspectives on the future. Adv Drug Deliv Rev. 158:4–16.
152.vChen Y, Chen H, Shi J (2013) In vivo bio‐safety evaluations and diagnostic/therapeutic applications of
chemically designed mesoporous silica nanoparticles. Adv Mater. 25(23):3144–76.
153. Wankhede M, Bouras A, Kaluzova M, Hadjipanayis CG. (2012) Magnetic nanoparticles: an emerging technology for malignant brain tumor imaging and therapy. Expert Rev Clin Pharmacol. 5(2):173–86.
154. Carter N, Martin-Misener R, Kilpatrick K, Kaasalainen S, Donald F, et al. (2010) The role of nursing leadership in integrating clinical nurse specialists and nurse practitioners in healthcare delivery in Canada. Nurs Leadersh. 23 (Spec No):167-85.
155. Naz S, Gul A, Zia M. (2020) Toxicity of copper oxide nanoparticles: a review study. IET nanobiotechnology. 14(1):1–13.
156.nLin L, Wang S, Deng H, Yang W, Rao L, et al. (2020) Endogenous labile iron pool-mediated free radical generation for cancer chemodynamic therapy. J Am Chem Soc. 142(36):15320–330.
157. Islam MM, Shahruzzaman M, Biswas S, Sakib MN, Rashid Tu. (2020) Chitosan based bioactive materials in tissue engineering applications-A review. Bioact Mater. 5(1):164–83.
158. Zhu T, Mao J, Cheng Y, Liu H, Lv L, et al. (2019) Recent progress of polysaccharide‐based hydrogel interfaces for wound healing and tissue engineering. Adv Mater Interfaces 6(17):1900761.
159. Xiao W, Gao H. (2018) The impact of protein corona on the behavior and targeting capability of nanoparticle- based delivery system. Int J Pharm. 552(1–2):328–39.
160. Soppimath KS, Aminabhavi TM, Kulkarni AR, Rudzinski WE. (2001) Biodegradable polymeric nanoparticles as drug delivery devices. J Control release. 70(1–2):1–20.
161. Banik B, Surnar B, Askins BW, Banerjee M, Dhar S. (2019) Dual-targeted synthetic nanoparticles for cardiovascular diseases. ACS Appl Mater Interfaces. 12(6):6852–62.
162. Cheng N, Bai X, Shu Y, Ahmad O, Shen P. (2021) Targeting tumor-associated macrophages as an antitumor strategy. Biochem Pharmacol. 183:114354.
163. Adityan S, Tran M, Bhavsar C, Wu SY. (2020) Nano-therapeutics for modulating the tumour microenvironment: Design, development, and clinical translation. J Control Release. 327:512–32.
164. Demirbolat GM, Altintas L, Yilmaz S, Degim IT. (2018) Development of orally applicable, combinatorial drug–loaded nanoparticles for the treatment of fibrosarcoma. J Pharm Sci. 107(5):1398–07.
165. Anselmo AC, Mitragotri S. (2016) Nanoparticles in the clinic. Bioeng Transl Med. 1(1):10– 29.
166. Singh AV, Ansari MHD, Rosenkranz D, Maharjan RS, Kriegel FL, et al. (2020) Artificial intelligence and machine learning in computational nanotoxicology: unlocking and empowering nanomedicine. Adv Healthc Mater. 9(17):1901862.
167. Zarrintaj P, Saeb MR, Stadler FJ, Yazdi MK, Nezhad MN, et al. (2022) Human Organs‐on‐Chips: A Review of the State‐of‐the‐Art, Current Prospects, and Future Challenges. Adv Biol. 6(1):2000526.
168. Ganeshkar MP, Mirjankar MR, Shivappa P, Gaddigal AT, Goder PH, et al. (2023) Biogenic synthesis of selenium nanoparticles, characterization and screening of therapeutic applications using Averrhoa carambola leaf extract. Part Sci Technol. 1–13.
169. Mukherjee S, Madamsetty VS, Bhattacharya D, Roy Chowdhury D, Paul MK, et al. (2020) Recent advancements of nanomedicine in neurodegenerative disorders theranostics. Adv Funct Mater. 30(35):2003054.
170. Fidler IJ. (1986) Rationale and methods for the use of nude mice to study the biology and therapy of human cancer metastasis. Cancer Metastasis Rev. 5:29–49.
171. Guimarães PPG, Gaglione S, Sewastianik T, Carrasco RD, Langer R, et al. (2018) Nanoparticles for immune cytokine TRAIL-based cancer therapy. ACS Nano. 12(2):912-31.
172. Yan L, Shen J, Wang J, Yang X, Dong S, et al. (2020) Nanoparticle-based drug delivery system: a patient-friendly chemotherapy for oncol ogy. Dose-Response. 18(3):1559325820936161.